Chapter 7
On The Eve of Shuttle ( 1973-1980)
While Skylab was being built, other events significant to the future of space exploration were taking place. The initiatives bore the imprint of Thomas O. Paine, acting administrator after Webb's resignation in 1968 and administrator of NASA from March 1969 until he returned to industry in September 1970. One goal was a broad approach to increased cooperation in space exploration. As had so many of our international space initiatives in the postwar period, this effort offered separate proposals to the Soviet Union and to Western European countries. The approach to the Soviet Union began in 1968, with suggestions for advanced cooperation, especially in the expensive arena of manned spaceflight. One area of Soviet vulnerability might be rescue of astronauts and cosmonauts. By now the Soviet Union had lost four cosmonauts in flight, three in one accident, one in another. They had always evidenced a singular concern for cosmonaut safety. Perhaps some joint program could develop a system of international space rescue. The dynamics seemed right; by 1969 the evidence was clear that, whether the Soviet Union had in fact been in a moon-landing race with the United States, the United States was ahead. Secrecy in space was virtually nonexistent; size of payloads, destinations of missions, performance —all were detectable by tracking systems.
Paine's first offer was for Soviet linkup with the Skylab orbital workshop. But the very hardware implied inequity. The Soviets were not interested. Further explorations found lively Soviet interest in a completely new project to develop compatible docking and rescue systems for manned spaceflight. Negotiations proceeded rapidly. Completed by George M. Low, acting administrator after Paine's departure, the grand plan for the Apollo-Soyuz Test project (ASTP) called for a mutual docking and crew exchange mission that could develop the necessary equipment for international rescue and establish such criteria for future manned systems from both nations. A Soyuz spacecraft would lift off from the Soviet Union and establish itself in orbit. Then an Apollo spacecraft would be launched to rendezvous and dock with the Soviet craft. Using a specially developed docking unit between the two spacecraft, they would adjust pressurization differences of the two spacecraft and spend two days docked together, exchanging crews and conducting experiments. All of this was agreed to and rapidly became a significant test for the validity of the detente agreements which President Richard M. Nixon had negotiated with the Soviet Union.
An unprecedented detailed cooperation between the two superpowers ensued. A series of joint working groups of Soviet and American specialists met over several years to work out the various hardware details and operational procedures. At the Nixon-Brezhnev summit in 1973, the prospective launch date was narrowed to July 1975. The most concrete example of U.S.-U.S.S.R. cooperation in space proceeded with good faith on both sides. The mission flew as scheduled on 15 July and smoothly fulfilled all objectives.
The Space Shuttle
The other major initiative of Paine's began on the domestic front and then expanded to the international arena. Skylab having been narrowed to the point that it would be a limited answer to the future of manned spaceflight, President Nixon appointed a Space Task Group to recommend broad outlines for the next 10 years of space exploration. Within this group, Paine won acceptance for the concept of the Space Shuttle. In its original conception, the Space Shuttle would have been a rocket-boosted airplane-like structure that would take off from a regular airport runway, fly to orbital speed and altitude, deploy satellites into orbit, repair or retrieve satellites already in orbit, and, using an additional Space Tug stage, lift manned and unmanned payloads throughout the solar system. Compared to earlier methods, the big changes would be that the launcher and Shuttle would be reusable for up to 100 flights, halving the cost per pound in orbit. But subsidiary changes were only slightly less important: satellites could be designed for orbital rigors, not the additional ones of rocket launch. In a manned mission, the Shuttle would handle a crew of up to seven people in orbit; three of these could be non-pilot scientists who went along to operate their experiments in an unpressurized laboratory carried in the Shuttle cargo bay. The flight crew alone could deliver 65,500 pounds of assorted satellites into orbit.
The Space Task Group submitted its report to the President on 15 September 1969. It offered three levels of effort: option 1 would feature a lunar-orbital station, an Earth-orbital station, and a lunar surface base in the 1980s; option 2 envisioned a Mars manned mission in 1986; option 3 included initial development of space station and reusable shuttles but would defer landing on Mars until some time before the end of the century. Eventual peak expenditures on these options were estimated to vary from $10 billion down to $5 billion per year. Study and rework went on for more than two years. Paine left NASA to return to industry; his successor, James C. Fletcher, took office in April 1971 and immediately reviewed the status of the Space Shuttle, particularly for its political salability. He became quickly convinced that the Shuttle as then envisioned was too costly to win approval. Total costs for its development were estimated at $10.5 billion. Fletcher instigated a rigorous restudy and redesign which cut the cost in half, mainly by dropping the plan for unassisted takeoff and substituting two external, recoverable, reusable solid rockets and an expendable external fuel tank. This proved to be salable; President Nixon approved the development of the Space Shuttle on 5 January 1972.
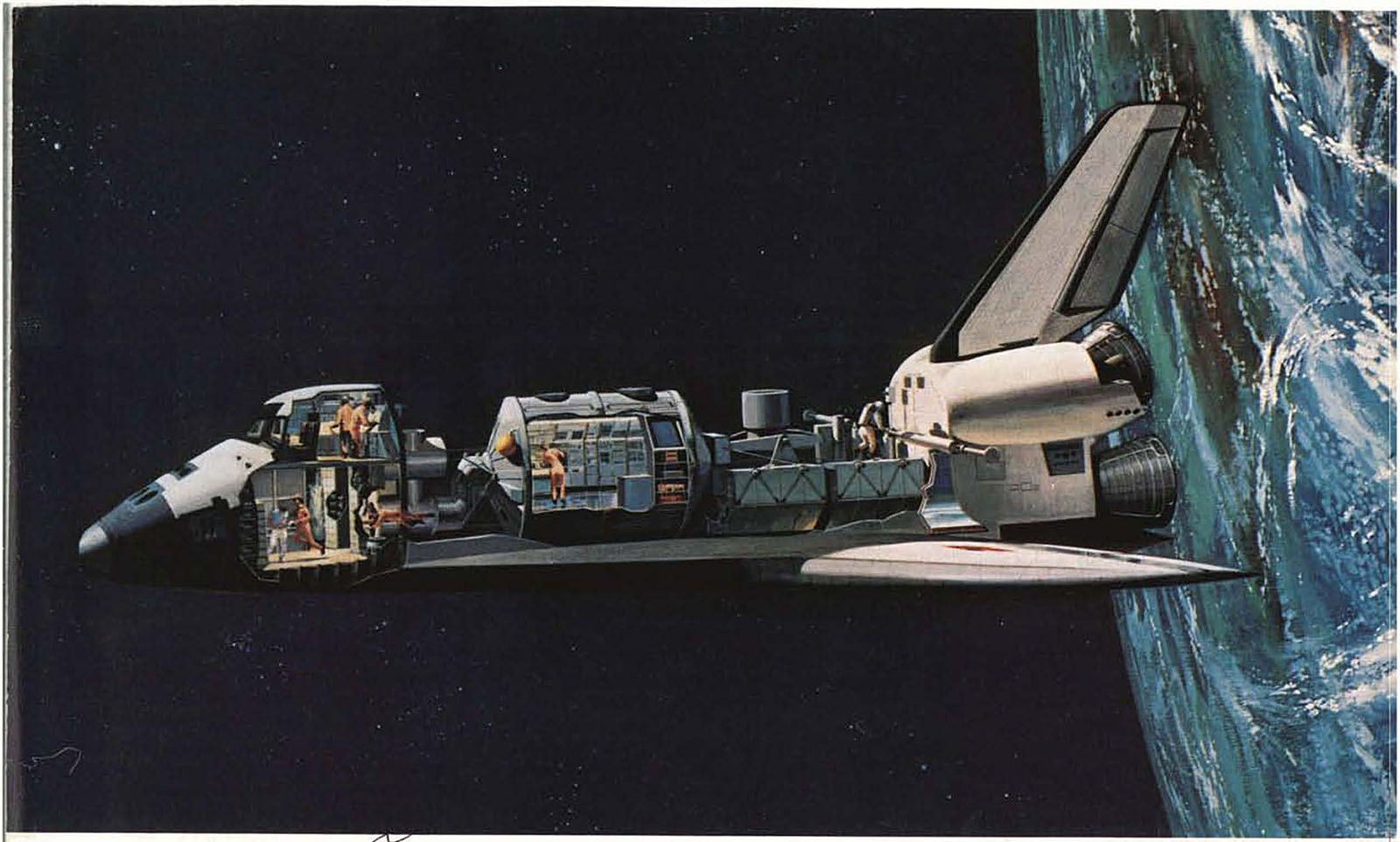
First Paine and then Fletcher had been trying to get a commitment from Western European nations for a major system in the Shuttle. Their own joint space program had not been an unqualified success. In 1964, Western European nations had joined to form two international space organizations, ELDO to produce launch vehicles and ESRO to produce spacecraft and collect and interpret results. The technical capability was there, but issues of assigning specific contracts to separate countries and allocating budgets hampered rapid European progress. A proposed booster had three stages, each developed in a different country. The launch record was a gloomy history of one kind of failure after another. After years of effort, Western Europe had little to show for its independent launch vehicle. On the other hand, much had been learned about multinational coordination of advanced technology, and successful joint projects like Concorde and several multinational military aircraft ventures (such as the Pavavia Tornado) had promoted a sophisticated aerospace community in Europe. Moreover, using American boosters, the ESRO group had successfully launched a variety of scientific satellites, applications satellites, and space probes. In addition to experienced contractors, the European space organizations had developed international centers, like ESTEC in the Netherlands, to carry out research and maintain ongoing management of space projects. By the early 1970s, there was general agreement on the need for a new, unified organization. Based on the strengthening capabilities of its aerospace community, the European Space Agency (ESA) was established in 1975. A new start was in the air.
It was into this restive environment that Paine came to talk about the next generation of the U.S. space program and to hold out promise of some discrete major segment to be developed and produced in Europe —a partnership that would give them a meaningful piece of the action with full pride of useful participation. Europe's response was warm, though it took a while to coalesce. Finally the joint decision was made: Western Europe agreed to build the self-contained Spacelab that would fit in the cargo bay of the Shuttle spacecraft; a pressurized module would provide a shirtsleeve environment for scientists to operate large-scale experiments; an unpressurized scientific instrument pallet would give large telescopes and other instruments direct access to the space environment. The cost, an estimated $370 million. In 1975 Canada joined the international effort, agreeing to foot the $30 million R&D bill for the remote manipulator used to emplace and retrieve satellites in orbit.
The Space Shuttle promised a whole new way of spaceflight: nonpilots in space; multiple payloads that could be placed where they were wanted or picked up out of orbit; new designs of satellites, free from the expensive safeguards against the vibrations and shocks of launch by rocket. The $5.2 billion program would buy two prototypes for test in 1978 and 1979. Projected flight programs from 1980 to 1991 identified a total of almost 1000 payloads to be handled by the Shuttle.
The largest consumer of the NASA budget and of management attention during the late 1970s was the Space Shuttle. Since its beginnings in the early 1970s, the development story for the Space Shuttle had been quite different from that of Apollo in the 1960s. The original projected costs had been halved to win the necessary political approval of the program; this cut was only achieved by making severe compromises in the original design —from a system that would take off from a runway like an airplane, fly into orbit, and return to land on a runway like an airplane, to a system that would take off vertically like a rocket, jettison the boosters and fuel tanks, and return to land on a runway like an airplane. This initial compromise was not to be the last, as the budget continued to be lean year after year. Potential development problems were worked around because the money was not available to investigate them. The consequences of this insufficient level of research during the development cycle were not apparent in the years when the Shuttle was being designed and the components fabricated. As late as 1977, when the orbiter Enterprise was carried aloft by a modified Boeing 747 and dropped to make approach and landing flights at Dryden Flight Research Center, progress was seen to be sure, if a little slow.
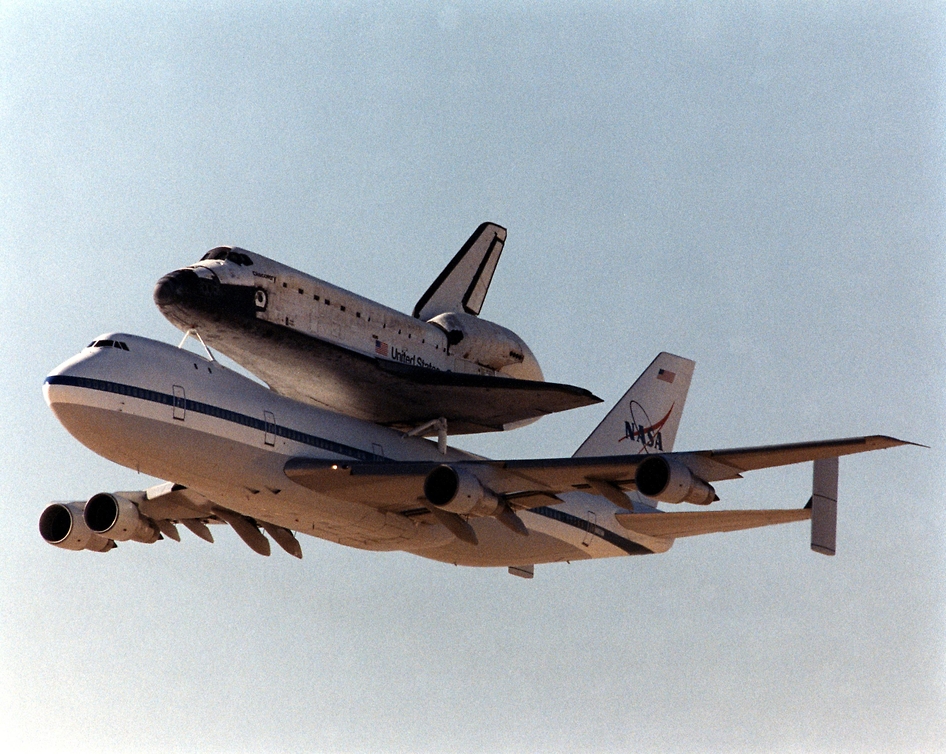
In 1978 it became obvious that serious problems were dogging the main engines. A cluster of three of these high-pressure liquid-hydrogen-fueled engines would propel the orbiter into orbit, aided by two solid-rocket boosters. Not only were the main engines expected to produce the highest specific impulse of any rocket engine yet flown, but they also had to be throttleable and reusable —to fire again and again for many flights before being replaced. By 1979, a series of painstaking component-by-component analyses had identified and fixed most of the problems and individual engines were experiencing better test runs; but the first firings of the clustered engines generated a new set of problems. Grudgingly these too yielded to concentrated engineering rework; by the end of 1980 the total requirements of 80,000 seconds of test firing was in hand.
The other pacing item on the orbiter was the thermal protection tiling that would shield most of the orbiter surface from the searing heat of reentry. Manufacture and application of the 33,000 tiles lagged so badly that early in 1979 NASA decided to ferry the orbiter from the manufacturer's plant in California to Kennedy Space Center so that the remainder of the tiles could be applied there while other work and system checks were being done. But problems continued. The tiles were brittle and easily damaged; they did not bond to the metal properly and thousands had to be reapplied; they were too fragile and thousands more had to be removed, made more dense, and reapplied. Between the tiles and the engines, the Space Shuttle budget overran for several years and the date for the first flight slipped two painful years, with serious consequences for many government, domestic, and international customers. By the end of 1980, however, first flight in the spring of 1981 seemed truly possible. Operational flights were solidly booked out to the middle of the 1980s and the other three orbiters were moving through manufacturing.
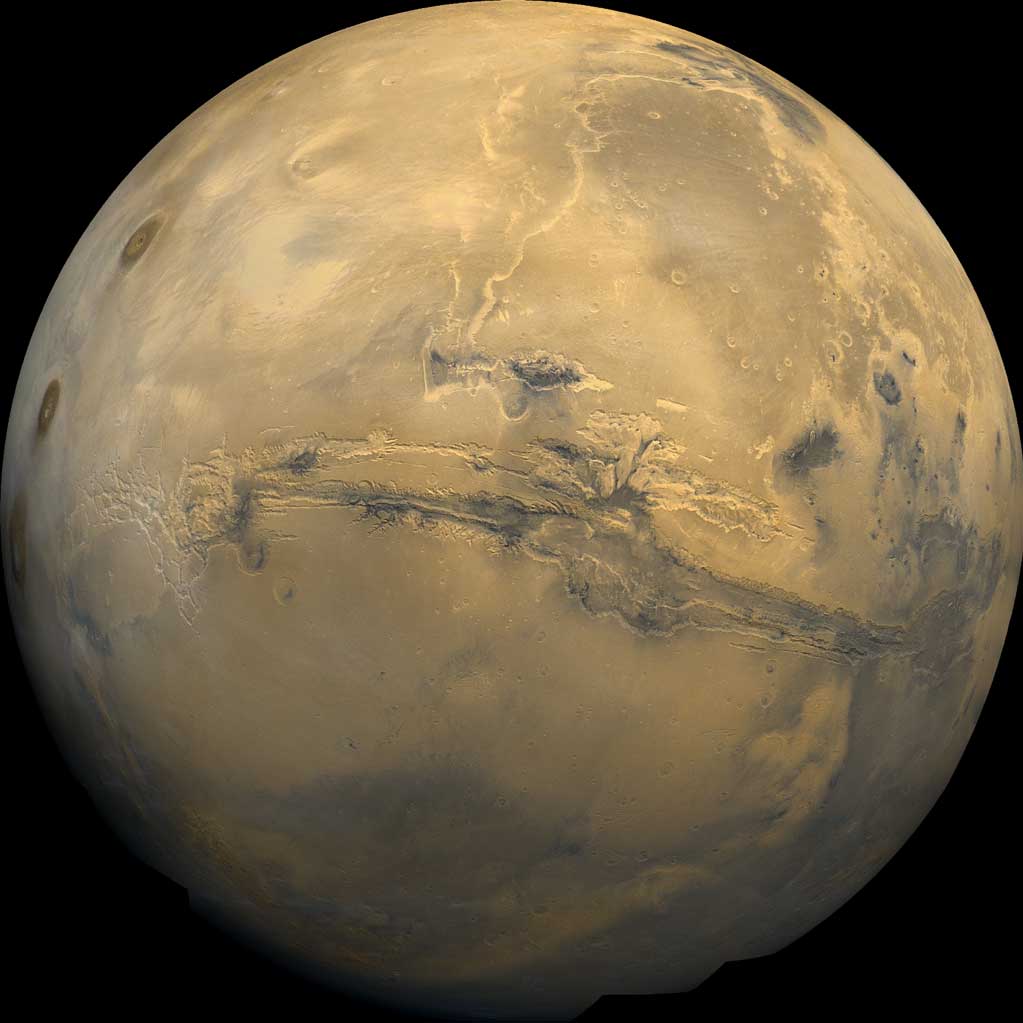
The Planets
In space science the big program was Viking, which represented the first major fruit of a decision NASA had made some years before: to focus the space science program on the planets. Apollo, the reasoning went, would keep scientists busy for years analyzing the mass of data and samples that had been returned from the Moon. Not until that information had been assimilated would there be a need to consider whether more information was needed from the Moon and, if so, what kind.
Meanwhile space science, while not neglecting the study of the Sun and the universe, would concentrate on the inner planets of our solar system and begin an assault on the enigmatic outer planets. Apollo had shown, and the early planetary flights had confirmed, that every celestial body had worthwhile lessons to teach — lessons that were important in their own right as science as well as lessons that illuminated problems on Earth. Why did Earth have the kinds and proportions of minerals that it had? Why tectonic plates and volcanism? Why oceans and the unique atmosphere of Earth? Why did our atmosphere circulate and transfer heat the way it did? Every new body we studied represented a new laboratory and a different set of data.
So it was that Mars, the most likely of the inner planets, became the first target of the more ambitious planetary program. In two launches the Viking program proposed to deploy four spacecraft in the vicinity of Mars; two orbiters would photograph the surface and serve as communications relays, while two landers would descend to the martian surface and photograph the terrain, measure and monitor the atmosphere and climate, and conduct chemical and biological tests on the soil for evidence of rudimentary life forms. It was very ambitious technology and complex science to be operated from over 40 million miles distance. But perform Viking did, in a technological triumph equal to (and in some ways greater than) the Apollo landings on the Moon. Arriving in the vicinity of Mars in mid-1976, the spacecraft went into orbit around the planet. Subsequently the two landers arced down to the rock-strewn surface where each landed safely. The two orbiters circled the planet, mapping most of the surface. That surface depicted by the orbiters, plus the weather and seismic reports from the landers, told a story of a planet with a quiescent present but a very different, active past. Volcanoes half again as high as any on Earth and great eroded canyons deeper and longer than any on Earth spoke of times, probably three billion years ago, when Mars was very active volcanically, with widespread liquid flows. Trace gases in the present thin atmosphere indicated a much denser atmosphere in the past. There was water, frozen in the polar ice caps; there were occasional dust storms; there were seasonal as well as diurnal variations in temperature; there was only a trace of seismic activity now. Viking's elaborate biology instruments detected no evidence of life forms. When the intensive one-year study of the planet ended, the spacecraft continued observations and reporting at intervals, providing further data on surface features, climate, and weather.
Earth's nearest planetary neighbor, Venus, was also probed during the last half of the 1970s. Two Pioneer spacecraft were launched toward Venus in the summer of 1978. Studying Venus presented a notably different problem than Mars or Earth. Its thick, heavy, hot atmosphere was impervious to normal photography and could be "seen" through only by means of radar. The first spacecraft arriving at Venus in December 1978, therefore, was an orbiter equipped with mapping radar to delineate the major features on the surface. The second spacecraft was a bus which released four probes in a broad pattern; these parachuted slowly through the atmosphere, sending back measurements until they crashed. The venusian atmosphere, they reported, was remarkably similar in composition and temperature on the day and night sides. There was a high sulfur content, with oxygen and water vapor at lower levels. By 1980 the orbiter had mapped over 80 percent of the venusian surface. Major features resembled two continents and a massive island chain—except there was no ocean. Instead a rolling plain enveloped the planet. One continent and the island chain were in the northern hemisphere. The continent was the size of Australia and had mountains taller than Everest; the island chain was apparently composed of two massive shield volcanoes more extensive than the Hawaii-Midway complex. The continent in the southern hemisphere was about half the size of Africa and exposed the lowest elevations on Venus in the Great Rift Valley, a huge trench 174 miles wide and 1395 long, with a depth similar to the great rift on Mars.
Study of the outer planets using more sophisticated spacecraft began in 1977 with the launch of Voyager 1 and 2 on 18-month flights to Jupiter. The Voyager system, Science magazine reported, was improved by a factor of 150,000 times over the Mariner 4 system, which flew to Mars in 1965. Voyager 1 made its closest approach to Jupiter in March 1979, with Voyager 2 following in July. The sensors recorded in fine-grain detail the intricate weather patterns on Jupiter and detected massive lightning bolts in the cloud tops. Passes by the Galilean moons revealed startling differences; active volcanoes on Io, ancient rings on Callisto marking the edges of huge impact craters. Europa's surface was laced with cracks from crustal movement, and Ganymede had a varying grooved and cratered surface.
With a boost from Jupiter's gravitational field, the Voyagers set course for distant, ringed Saturn, where Voyager 1 arrived in November 1980 and Voyager 2 arrived in August 1981. With sufficient control gas remaining, the mission extended to a faraway Uranus flyby in January 1986, with a Neptune flyby planned for August 1989. The venerable Pioneer II had visited Saturn in September 1979, discovering faint rings outside those discernible from Earth and demonstrating a safe flight path for Voyager 2 to follow on its path to Uranus.
In the study of the Sun and its interrelationships with Earth, NASA continued analysis of the mass of data acquired by Skylab's Apollo telescope mount. OSO 8, launched in 1975, to make a detailed study of the minimum phase of the 11-year solar cycle, returned data until 1978. Helios 2, part of a joint program with the Federal Republic of Germany to study the basic solar processes, was launched in 1976. As the solar cycle moved toward its maximum phase, the Solar Maximum Mission was launched in 1980 to study solar flares in the wavelengths in which the Sun releases most of its energy. Problems with the satellite led to rendezvous and retrieval by a Shuttle crew in 1984.
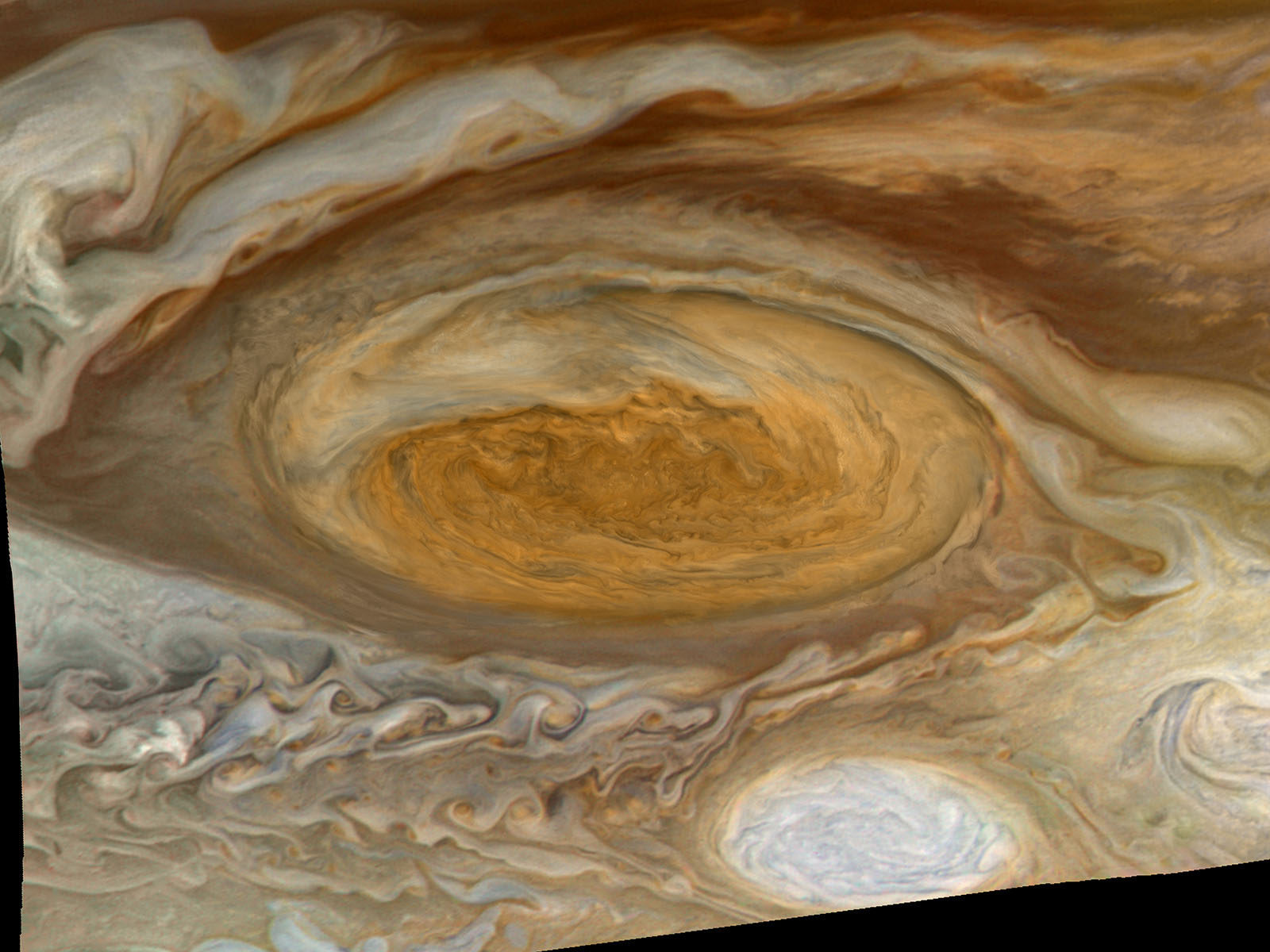
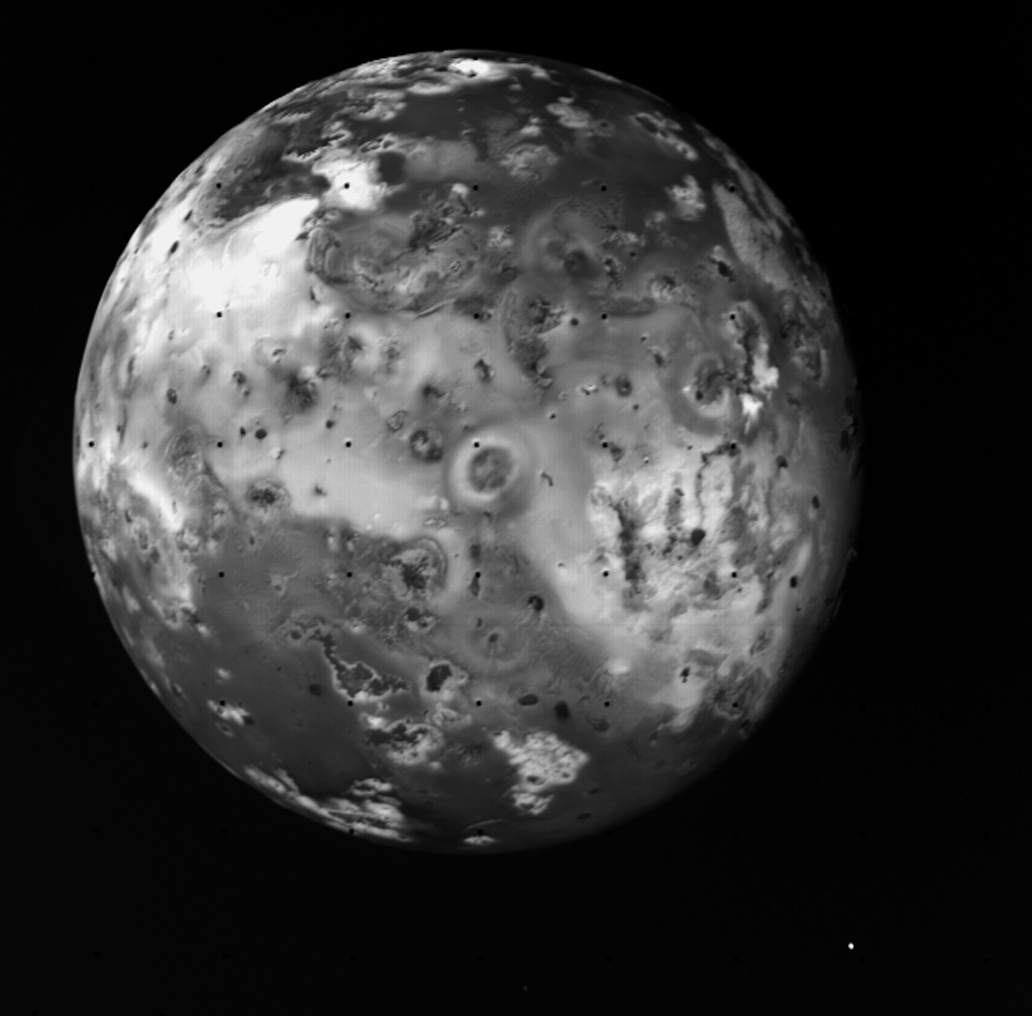
To study the effects of solar radiation on Earth's magnetosphere and atmosphere, NASA launched International Sun-Earth Explorer 1 and 2 in 1977. Positioned some distance apart but in similar elliptical orbits, the two satellites (one provided by NASA, the other by the ESA) monitored the complex interactions of Earth's magnetosphere with incoming solar radiation. In 1978 ISEE 3 was added to the system. Positioned much farther out from Earth, the spacecraft receives the solar wind and flares about an hour earlier, when they are unaffected by the magnetosphere.
In study of the universe, the major program of the second half of the 1970s was the series of three high-energy astronomy observatories. HEAO 1, launched in 1977 and the heaviest scientific satellite to date, surveyed the sky for x-ray sources, identifying several hundred new ones. HEAO 2, following the next year, studied in detail the most promising of those sources. HEAO 3, launched in 1979, surveyed the sky for gamma-ray sources and cosmic-ray flux. The other satellite orbited for study of the universe was the International Ultraviolet Explorer (IUE). Carrying instruments from NASA, the United Kingdom, and the ESA, IUE recorded ultraviolet emissions using two ground control centers from which the experimenters could direct the observations of the satellite much as is done with telescopes in observatories on Earth.
An intensified activity for NASA in the latter half of the 1970s was the congressionally mandated study of Earth's upper atmosphere, to learn more about the effects of gases such as freon on the ozone layer. A continuous measuring program resulted; several agencies provided data from which a detailed model of the complex processes could be constructed. The space applications program was active in the late 1970s. Communications research continued with the launch in 1976 of Communications Technology Satellite 1. A joint project with Canada, CTS 1, investigated the possibilities of high-powered satellites transmitting public service information to small, inexpensive antennae in remote locations.
Landsat 3 was launched in 1978, providing continuity for the flow of data to a growing number of users of Earth resources information. The most ambitious new Earth resources program was in agriculture. Encouraged by the results of the experimental Large Area Crop Inventory Experiment that ended in 1978 after demonstrating 90 percent accuracy in predicting the wheat production in the U.S. Southern Great Plains and U.S.S.R., the Department of Agriculture, with technical assistance from NASA and NOAA, began AgRISTARS (Agriculture and Resources Inventory Surveys Through Aerospace Remote Sensing).
A new form of resources surveying was attempted in 1978 with the launch of Seasat 1. Intended to report on such variables as sea temperature, wave heights, surface-wind speeds and direction, sea ice, and storms, Seasat was an instant success. Unfortunately its life was cut short after three months in orbit by electrical power failure. Enough data had been recorded, however, to verify the effectiveness of the instrumentation and the existence of a group of potential users in the weather, maritime, and fisheries communities.
In environmental research, NASA launched Nimbus 7 in 1978, the last of the series of large experimental weather satellites. One of its instruments, together with one on Nimbus 4 and the observations of SAGE (Stratospheric Aerosol and Gas Experiment, launched in 1979), provided a profile and model of the ozone layer. The nations's weather satellite system was augmented in 1978 by the launch of Tiros-N and NOAA 6, the first two of a new generation of improved weather satellites in near-polar orbit. Tiros-N was a principal U.S. contributor to the international Global Atmospheric Research Program.
In geophysical research, a small experimental Heat Capacity Mapping Mission satellite was launched in 1978 to derive day and night temperatures of rock formations as a possible means of locating mineral-bearing strata. In 1979 another small satellite, Magsat, went into low orbit to take finer scale readings of anomalies in Earth's magnetic field that are directly related to crustal structure and therefore to possible mineral deposits. In earthquake research, NASA completed in 1979 the fourth phase of data gathering along the San Andreas Fault in California. By means of satellites ranging from specified points along both sides of the fault, experimenters estimated that the tectonic plates were moving 2.4 to 4.8 inches per year.
Aircraft and the Environment
In keeping with rising energy concerns of the 1970s, NASA committed considerable resources to new engine and aircraft technologies to increase flight efficiency as a means of conserving fuel. The Aircraft Energy Efficiency program was begun in 1975 to develop fuel-saving techniques that would be applicable to current aircraft as well as future designs. The project covered several areas of investigation: more efficient wings and propellers; composite materials that were lighter and more economical than metal; improved fuel efficiency in jet engines; new engine technologies for aircraft in the future.
The super critical wing was only one aspect of activity that also led NASA into the arcane subject of laminar flow-control. A smooth flow of air over the surface of a plane, or laminar flow, is a characteristic of low speeds. At cruising speeds, the air flow becomes turbulent, creating increased drag. Using models and analytical testing, NASA developed a system of tiny holes on the wing surface and a lightweight suction system to draw off the turbulent air. By the late 1980s, the agency was ready to begin flight testing of a laminar flow-control system for possible use on commercial aircraft.
Other research efforts were carried out through the Engine Component Improvement Program. The objective was to target engine components for which wear and deterioration led directly to decreased fuel efficiency in jet engines. As a result, new components to resist erosion and warping were introduced, along with improved seals, ceramic coatings to improve performance of gas-turbine blades, and improved compressor design. Research results were so positive and so rapidly adaptable that new airliners of the early 1980s like the Boeing 767 and McDonnell Douglas MD-80 series used engines that incorporated many such innovations.
For business jets, NASA rebuilt an experimental turbofan, incorporating newly engineered components designed to reduce noise. Completed by 1980, this project successfully developed engines that generated 50 to 60 percent less noise than current models. For larger transports, Lewis Research Center started tests of two research engines that cut noise levels by 60 to 75 percent and reduced emissions of carbon monoxide and unburned hydrocarbons as well.
In a different context, NASA became engaged in procedures for flight operations in increasingly congested air space. Among the issues that needed assessment were aircraft noise during landing and takeoff over populated areas; safe approach and landing procedures in bad weather; and methods for controlling high-density traffic patterns. Useful information emerged from a modified Boeing 737 twin-jet transport. In the plane's passenger area, NASA technicians put together a second cockpit equipped with the latest innovations in instrumentation. This second cockpit became the flight center for research operations; the crew occupying the standard cockpit in the 737's nose functioned as a backup. In addition to precision descent and approach procedures on instruments, the plane played a key role in demonstrating the Microwave Landing System in 1979. The International Civil Aviation Organization eventually adopted the Microwave Landing System over a competing European design to be used as the standard system around the world.
At Ames, scientists became interested in using aircraft as platforms for investigations of terrestrial as well as astronomical phenomena. Beginning in 1969, Ames acquired a number of different research planes and launched several imaginative investigations that continued over the following decades. High-altitude missions relied on a pair of Lockheed U-2 aircraft, originally supplied to the Air Force as reconnaissance planes. They carried out Earth resources observations, compiled land usage maps, surveyed insect infested crops, and measured damage from floods as well as forest fires. The highflying U-2 aircraft provided information covering hundreds of square miles; for a more intensive look at details in a smaller area Ames brought in other specialized planes that flew mid-altitude missions.
One of the pioneers in mid-altitude missions was a refurbished airliner—a Convair 990 christened the Galileo. Commencing operations in the early 1970s, the four-engine jet conducted a variety of tasks, such as infrared photography, detection of forest fires, and meteorological investigations. Over the Bering Sea in 1973, a joint study with the Soviet Union gathered data on meteorological phenomena, ice flow, and wildlife migratory patterns. The first Convair was lost in a tragic midair collision with a Navy patrol plane, but its operations had been so productive that acquisition of a second plane was authorized, and Galileo II went to work in 1974. Conducting research at mid-altitude heights, the new Convair 990 made international missions as well, including archaeological studies of Mayan ruins and observations of monsoon patterns in the Indian Ocean.
Other planes were added, like the small Learjet and the huge Lockheed C-141 Starlifter, which became operational with the Ames fleet in 1974. The Starlifter's interior size and load-carrying capacity made it the best candidate for installation of a 915-centimeter telescope for astronomical observations. Many of the C-141 missions, as well as those involving other Ames research planes, were international in scope. In 1977, the C-141, known as the Kuiper Airborne Observatory, flew to Australia to make observations of the planet Uranus during especially favorable astronomical conditions. American and Australian scientists studied the planet's atmosphere, composition, shape, and size, and discovered that Uranus possessed equatorial rings.
At about the same time, the Learjet, equipped with a 30-centimeter infrared telescope, was operating high over the Arctic on a different international mission. Known as Project Porcupine, Ames worked with the Max Planck Institut fur Physik und Astrophysik in a study of the coupling between the magnetosphere and the ionosphere. The experiment called for the launch of a sounding rocket from Sweden. After the rocket ejected a barium charge, the Learjet followed the barium trail along the Earth's magnetic lines of force. Collectively, these researches by aircraft on a global scale enhanced professional contacts for NASA personnel and generated favorable foreign press coverage for the agency as well as for the United States.
As Ames proceeded to carve out its niche in using aircraft as research platforms, the center also strengthened its role in flight research, moving beyond wind tunnel testing to flight testing. Taking advantage of Congressional support for aeronautical research, the director of Ames, Hans Mark (appointed 1969), guided the center into research on short-haul aircraft, including V/STOL designs. Since the mid-1960s, Ames had been working with the U.S. Army on helicopter research, relying on the big lowspeed tunnels at Ames, along with its excellent simulator equipment and other facilities. By the 1970s, both the FAA and the Air Force were working with Ames on a new generation of short-takeoff transports. In 1976, to the chagrin of Langley, Ames officially became NASA's lead center in helicopter research. Although the Pioneer project and future planetary missions shifted to the JPL at the same time (completed by 1980), the new aircraft programs enlivened activities at Ames.
Among the rotor craft investigations, one of the most interesting involved the XV-15 Tilt Rotor Research Aircraft, with wingtip-mounted engines. For takeoff and landings, the engines remained vertical, with the big rotors providing lift; once in the air the engines and rotors tilted to the horizontal, propelling the XV-15 forward. Bell Helicopter Textron built two aircraft for NASA and the Army. The first XV-15 went to Ames in 1978 for extensive tests in the 40 x 80 foot wind tunnel, to be followed by flight tests at Bell's plant in Texas. The first demonstration of inflight tests of the two prototypes was underway at Ames and at Dryden Flight Research Center during 1980.
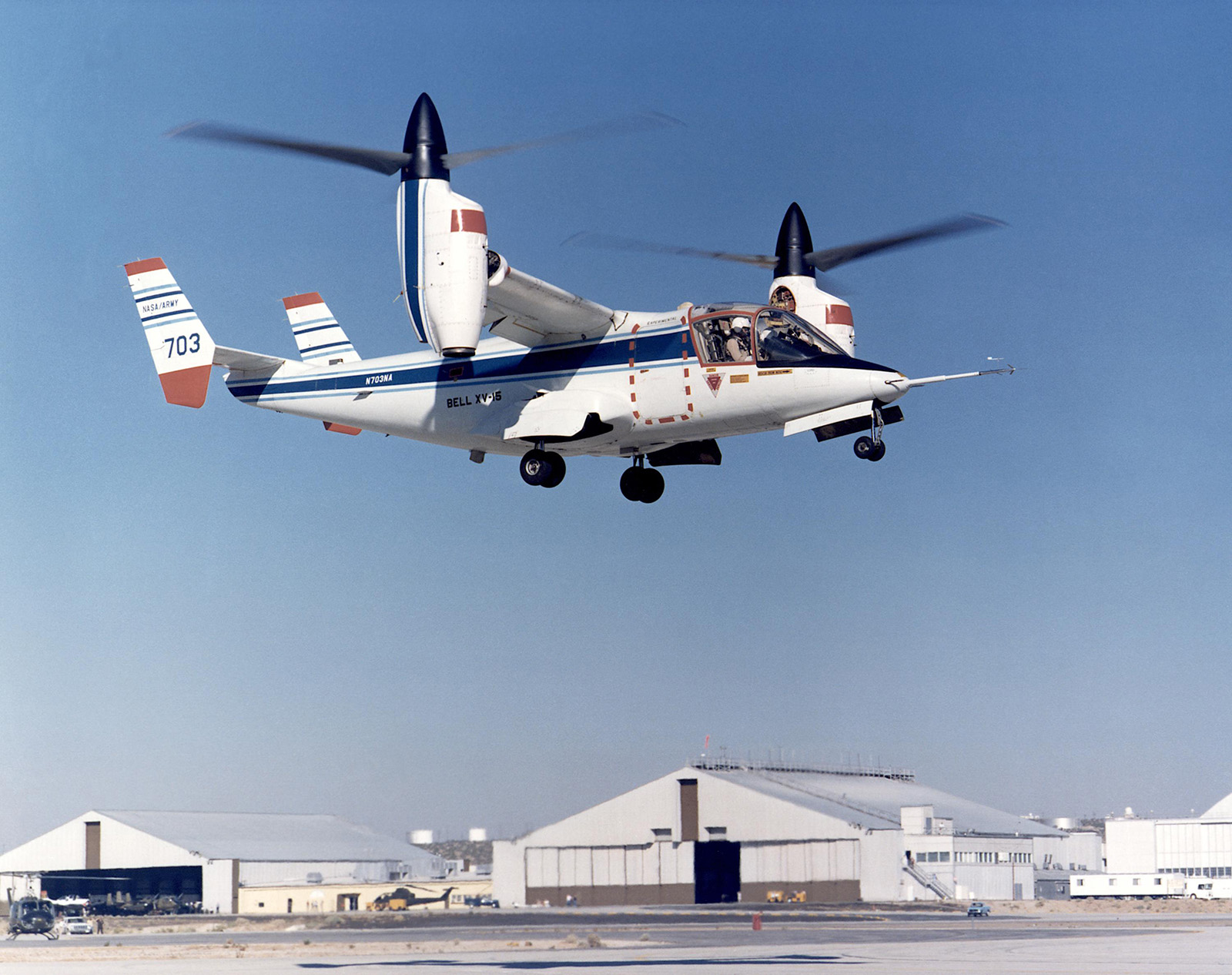
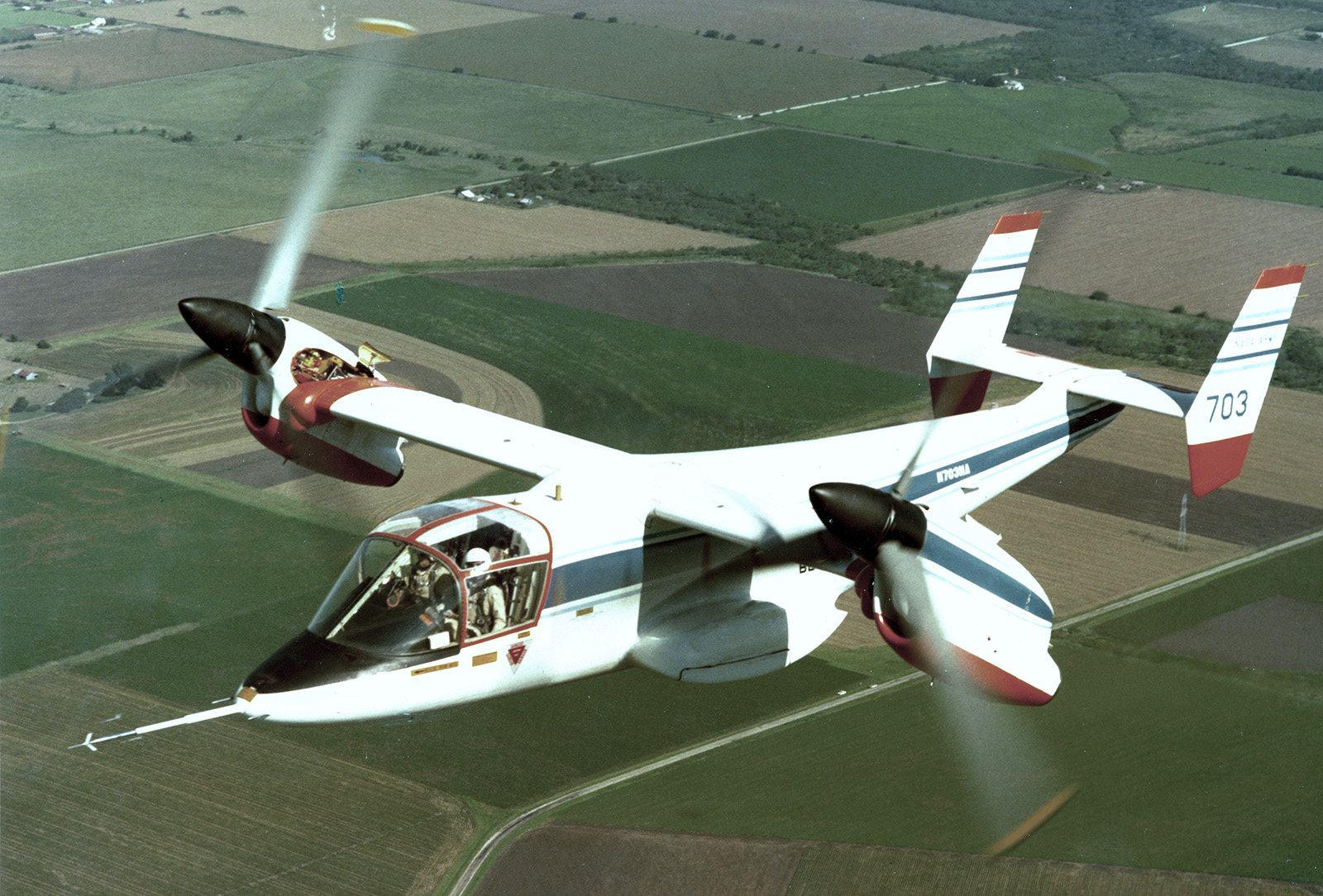
Somewhat more conventional was the Quiet Short-Haul Research Aircraft to investigate new technologies for commercial airliners. The research plane was a hybrid, using an extensively modified de Havilland C-8A Buffalo. Under contract to NASA, Boeing rebuilt the plane with new avionics, new wings and tail, and a quartet of jet engines mounted above the wing to generate "upper surface blowing" in order to increase lift. The plane made its maiden flight at Boeing's Seattle plant in 1978, then flew to Ames for continued flight tests. The short takeoffs and quiet operations of the aircraft yielded much information for application in both civil and military design. One intriguing series of tests led to a successful landing and takeoff from an aircraft carrier— the first four-engine jet plane to accomplish this feat.
For NASA, the decade of the 1980s seemed particularly promising. Its aeronautical programs were successful; space science had seen solid achievements; and progress in the Space Shuttle raised confidence for prospects of outstanding missions to come. That confidence was to be severely tested.