Chapter 2
Exploring the Human Factor
(1948 - 1958)
THE development of the large liquid-fueled rocket made the dazzling prospect of manned flight beyond Earth's atmosphere and into the vacuum of space increasingly feasible from the standpoint of propulsion. By 1950, however, only instrumented sounding rockets, fired to ever higher altitudes in both the United States and the Soviet Union, had reached into space before falling earthward. Although a number of these experimental shots carried living organisms - everything from fungus spores to monkeys in the United States, mainly dogs in the U.S.S.R. - the data acquired from telemetry and from occasional recovery of rocket nose cones had not shown conclusively how long organisms could live in space, or indeed whether man could survive at all outside the protective confines of his atmosphere. Scientists still were hesitant to predict how a human being would behave under conditions to be encountered in space flight. Thus while space flight became technologically practicable, physiologically and psychologically it remained an enigma.
In the early 1950s an acceleration of efforts in upper-atmospheric and space medical research accompanied the quickened pace of rocket development in this country and in the Soviet Union. During the next few years medical specialists, profiting from substantial progress in telemetering clinical data, learned a great deal about what a man could expect when he went into the forbidding arena of space.1 Much of the confidence with which the engineers of Project Mercury in 1958 approached the job of putting a man into orbit and recovering him stemmed from the findings of hundreds of studies made in previous years on the human factors in space flight.
Since the National Advisory Committee for Aeronautics was interested almost exclusively in the technology of flight, research in the medical problems of space flight, like aviation medicine in previous decades, was the province primarily of the military services and of some civilian research organizations receiving funds from the military. Of the three services, the United States Air Force, rich in background in aeromedical research and assuming that space medicine was but an extension of aviation medicine, undertook most of the early inquiry into the psychophysiological problems of extra-atmospheric flight.
Beginnings of Space Medicine
After the Second World War the Air Force acquired the talents of a number of scientists who had done much remarkable research on the medical aspects of high-speed, high-altitude airplane flight for Germany's Luftwaffe.2 Most of these German physicians, physiologists, and psychologists were brought to the expanding Aeromedical Laboratory at Wright-Patterson Air Force Base, near Dayton, Ohio. Six of the more prominent German aeromedical specialists, Hubertus Strughold, Hans-Georg Clamann, Konrad Buettner, Siegfried J. Gerathewohl, and the brothers Fritz and Heinz Haber, were assigned as research physicians to the Air Force School of Aviation Medicine, located on the scrub prairies of south central Texas at Randolph Air Force Base, outside San Antonio. The commandant of the school was Colonel Harry G. Armstrong, author of the classic text in aviation medicine.3 While heavily instrumented V-2s lumbered upward from White Sands and plastic research balloons lifted seeds, mice, hamsters, fruit flies, and other specimens into the upper atmosphere, Armstrong and his associates were already considering the medical implications of flight by man into the hostile space environment.
In November 1948, Armstrong organized at Randolph a panel discussion on the "Aeromedical Problems of Space Travel." Featuring papers by Strughold and Heinz Haber and commentary by six well-known scientists from universities and the military, the symposium perhaps marked the beginning of formal, academic inquiry into the medical hazards of extra-atmospheric flight. Before this epochal gathering ended, Strughold had resolved the contradiction inherent in the title of the symposium by emphatically using the term "space medicine."4
The following February, Armstrong set up the world's first Department of Space Medicine, headed by Strughold and including the Habers and Konrad Buettner.5 In November 1951, at San Antonio, the School of Aviation Medicine and the privately financed Lovelace Foundation for Medical Research at Albuquerque, New Mexico, sponsored a symposium discreetly entitled "Physics and Medicine of the Upper Atmosphere." It was still not respectable to speak plainly of space flight within the Air Force, which only that year had cautiously reactivated its intercontinental ballistic missile project and remained sensitive to "Buck Rogers" epithets from members of Congress and the taxpaying public. A good portion of the material presented by the 44 speakers at the 1951 symposium, however, covered the nature of space, the mechanics of space flight, and the medical difficulties of sending a man beyond the sensible and breathable atmosphere.6
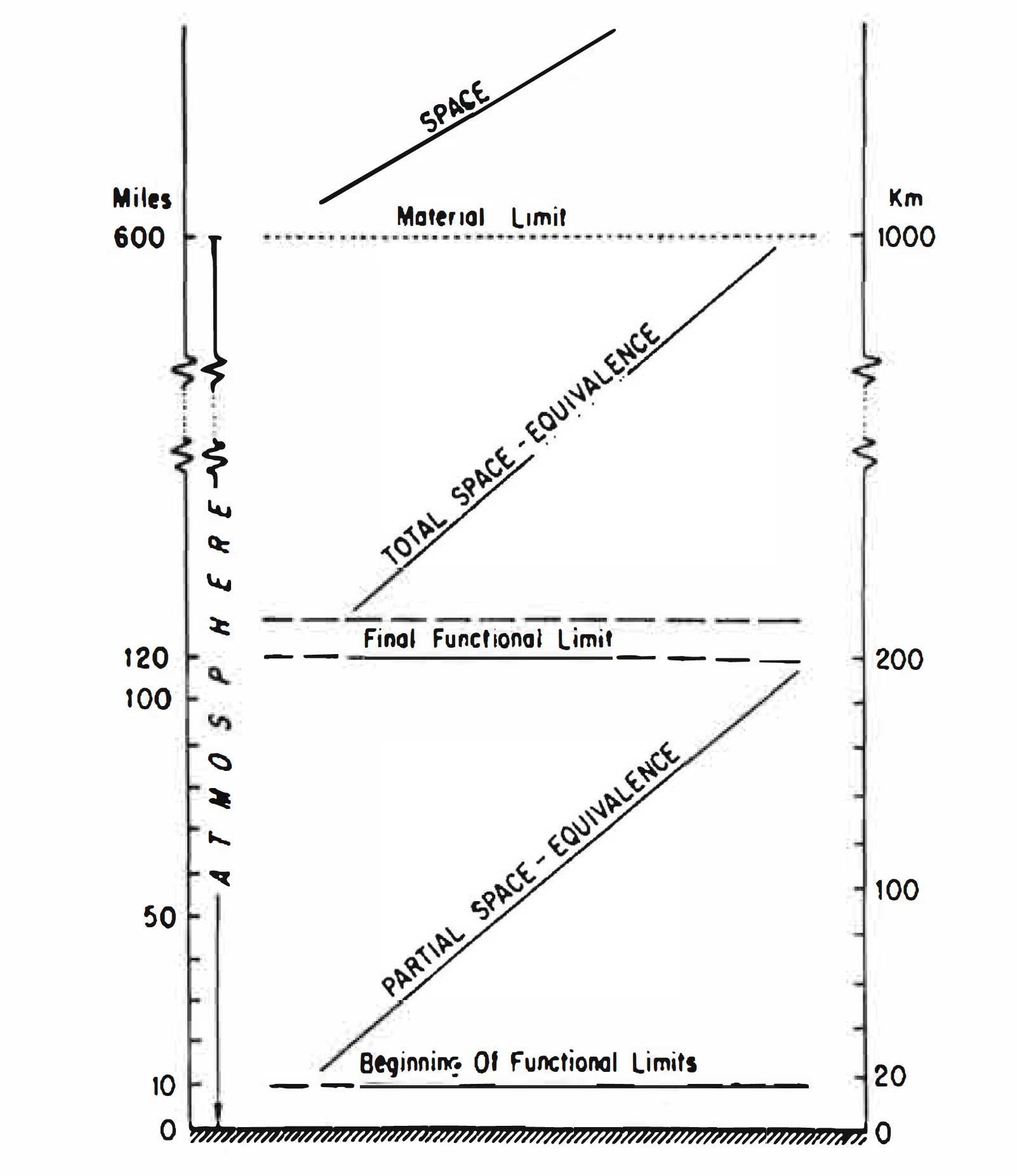
It was at this meeting that Strughold, later to acquire a reputation as the "father of space medicine," put forth what is perhaps his most notable contribution - the concept of "aeropause," a region of "space-equivalent conditions" or "atmospheric space equivalence." Strughold pointed out that while many astronomers, astrophysicists, and meteorologists set the boundary between the atmosphere and space at about 600 miles from Earth, the biological conditions of space begin much lower, at about 50,000 feet. Anoxia is encountered at 50,000 feet, the boiling point of body fluids at 63,000 feet, the necessity for carrying all respiratory oxygen within a manned compartment at 80,000 feet, meteoroids at 75 miles, and the darkness of the space "void" at 100 miles. Above 100 miles the atmosphere is imperceptible to the flyer. "What we call upper atmosphere in the physical sense," said Strughold, "must be considered - in terms of biology - as space in its total form." Hence manned ballistic or orbital flight at an altitude of 100 miles would be, for all practical purposes, space flight.7
The rocket-powered research airplanes of the postwar years, beginning with the X-1, the first manned vehicle to surpass the speed of sound, took American test pilots well into the region of space equivalence. On August 26, 1954, when Major Arthur Murray of the Air Force pushed the Bell X-1A to an altitude of 90,000 feet, he was above 90 percent of the sensible atmosphere. Two years later, in the more powerful Bell X-2, Air Force Captain Iven Kincheloe climbed to 126,000 feet, "a space-equivalent flight to a very high degree."8 The X-15, still on the drawing boards in the mid-fifties, was being designed to rocket its pilot to an altitude of 50 miles at nearly seven times the speed of sound. And human-factors research in the X-15 project, involving the development and testing of a new full-pressure flying suit, centrifuge conditioning to high acceleration forces, and telemetering a wide range of physiological data in flight, would contribute substantially to medical planning for space travel.9
Zero G
At peak speed and altitude an X-15 flight was supposed to afford about five minutes of "weightlessness" or "zero g." This is the effect created when a vehicle is balanced between centrifugal and centripetal forces - when the gravitational pull of Earth and other heavenly bodies is exactly balanced by the inertial character of the vehicle's motion. Weightlessness is undoubtedly the most fascinating medical characteristic of space flight, and it aroused the most speculation among aviation physicians in the late forties and early fifties. To be sure, approximations of zero g were not totally new human experiences; a common illustration of the sensation is the sudden partial lightening of the body in a rapidly descending elevator. But the necessity to function at zero g - to eat and drink, to eliminate body wastes, to operate the spacecraft controls - was a new requirement and presented new problems for the aeromedical teams.
Flight physicians were almost unanimous in expressing forebodings about the effect of weightlessness on man's physical and mental performance. Some feared that the body organs depended on sustained gravity and would not function if deprived of the customary gravitational force. Others worried over the combined effects of acceleration, weightlessness, and the heavy deceleration during atmospheric entry. Still other experts were concerned especially about perception and equilibrium. For example, Heinz Haber and Otto Gauer, another émigré German physician who joined the Air Force aeromedical program, noted t the brain receives signals on the position, direction, and support of the body from four mechanisms - pressure on the nerves and organs, muscle tone, posture, and the labyrinth of the inner ear. They theorized that these four mechanisms might give conflicting signals in the weightless state and that such disturbances "may deeply affect the autonomic nervous functions and ultimately produce a very severe sensation of succumbence associated with an absolute incapacity to act."10
The basic difficulty retarding the study of weightlessness was the impossibility of duplicating the exact condition on Earth. The X-15, considered by many in the mid-fifties to be the penultimate step to manned orbital flight, progressed slowly and would fly too late to shed much light on the problem of zero g for Project Mercury. By the fall of 1958, however, when the newly formed National Aeronautics and Space Administration undertook to orbit a manned satellite, American aeromedical researchers had been studying the gravity-free condition intensively for some eight years.
The best but most expensive device for zero-g experimentation was the sounding rocket. For several years, beginning with the V-2 firings from White Sands, parachutes for nose cones containing rocket-launched animals invariably failed to open and the subjects were killed on impact. The first successful recovery came in September 1951, when an instrumented monkey and 11 mice survived an Aerobee launch to 236,000 feet from Holloman Air Force Base, New Mexico. The last of three Aerobee shots at Holloman, in May 1952, like the previous experiments, carried a camera on board to photograph two mice and two monkeys under acceleration, weightlessness, and deceleration. An Air Force aeromedical team, headed by James P. Henry, a physician who later would direct the Mercury animal program, and young Captain David G. Simons, found no adverse effects on the animals.11
For the next six years the priority military ballistic missile program almost monopolized rocket development in the United States. Medical experimentation employing live test subjects launched to high altitudes by rockets came to a virtual standstill. By contrast, during the same period from 1952 to 1957, researchers in the Soviet Union carried out numerous animal rocket flights, with dogs of the Pavlovian sort being their favorite passengers. By late 1957, when the Soviets sent the dog Laika into orbit aboard Sputnik II, the peak altitude of their vertical launches of animals was nearly 300 miles, and the Russian scientists had perfected a technique for catapulting animals from nose cones and recovering them with parachutes. Apparently the Russians also were able to measure a wider range of physiological reactions than their American counterparts.12
During the six-year hiatus in animal rocket experimentation in this country, investigators had to resort to the aircraft, "the oldest aeromedical laboratory," for studying the weightless phenomenon.13 In 1950, Fritz and Heinz Haber, of the Air Force School of Aviation Medicine, had considered various ways of simulating zero g for medical experiments. Discarding the free fall and the elevator ride, the Habers concluded that the best technique involved an airplane flight along a vertical parabola, or "Keplerian trajectory." If properly executed, such a maneuver could provide as much as 35 seconds of zero g and a somewhat longer period of subgravity, a condition wherein the body is under only partial gravitational stress.14 During the summer and fall of 1951 test pilots A. Scott Crossfield of NACA and Charles E. Yeager of the Air Force tried out the technique, flying a number of Keplerian trajectories in jet interceptors. Up to 20 seconds of weightlessness resulted from some of these flights. Crossfield reported initial "befuddlement" during zero g but no serious loss of muscle coordination, while Yeager described a sensation of falling and in one instance of spinning and feeling "lost in space." The latter sensation the physicians and psychologists called "disorientation."15
The Habers' technique and these early experiments with it represented a promising beginning, but as one Air Force aeromedical specialist pointed out, "The results of these flights were inconclusive in many respects."16 An enormous amount of work remained before students of weightlessness could do much generalizing about this greatest anomaly of space flight.
In 1953 a small group comprising the Space Biology Branch of the Aeromedical Field Laboratory at Holloman Air Force Base inaugurated an ambitious program of parabolic flights to continue the investigations of weightless flight that had halted with the termination of the Aerobee animal launches in the spring of 1952. Supervised by Major David G. Simons, a physician who acted as test subject on many occasions, the Holloman studies for two years utilized T-33 and F-89 jet aircraft. Late in 1955, after Captain Grover J. D. Schock came to the field laboratory as task scientist, the standard tool for zero g research became the F-94C, which offered a longer parabola than other aircraft and thus a longer period of weightlessness. In the summer of 1958 the Air Force canceled all zero-g research at Holloman, and the coterie of scientists broke up. Colonel John P. Stapp, head of the field laboratory, and Simons went elsewhere, while Schock turned his attention to other research projects.17
For three years before the termination of the Holloman flight program, students of zero g at the School of Aviation Medicine had duplicated and even surpassed the investigations being carried out in New Mexico. Although sponsored by the Department of Space Medicine, the program carried out at Randolph Air Force Base was actually directed by Siegfried Gerathewohl, who was not a member of the department. Gerathewohl and his colleagues began their studies with the T-33 jet trainer, but like their counterparts in New Mexico, they soon turned to the superior F-94C. Major Herbert D. Stallings, a Randolph physician, estimated that by April 1958 he had flown more than 4,000 zero-g trajectories and compiled about 37 hours of weightless flight.18
Gerathewohl, Simons, Schock, and the other scientists at Randolph and Holloman tried to get as great a variety of information as possible during the 30 to 40 seconds of weightlessness and subgravity produced by the F-94C flights. They carried out numerous eye-hand coordination tests, for example, wherein a subject tried to make crosses in a pattern or hit a target with a metal stylus. Subjects usually missed their mark in the first moments of zero g or subgravity, but most of them improved their performance with their cumulative experience. The Air Force scientists also studied eating and drinking, bladder function, and disorientation after awakening during weightlessness; the functions at zero g of various animals, especially cats, whose vestibular organs had been removed; and the phenomenon called the "oculo-agravic illusion," wherein luminous objects seen in the dark appear to move upward during weightlessness.19
At the Wright Air Development Center, in Ohio, a team of researchers headed by Major Edward L. Brown picked up the experimental program discontinued at Holloman in mid-1958, except that they used the relatively slow, propeller-driven C-131 transport in their studies. A parabola in a C-131 gave only 10 to 15 seconds of weightlessness, but the spacious interior of the cargo carrier made it possible to observe the reactions of several subjects simultaneously, including their coordination and locomotion and even their ability to walk along the ceiling while wearing shoes with magnetic soles.20
In general the aeromedical specialists at Randolph, Holloman, and Wright-Patterson - as well as those in more modest programs at the Navy School of Aviation Medicine, Pensacola, Florida, and at the NACA Lewis Flight Propulsion Laboratory in Cleveland - found that the principal problems of weightless flight seemed solvable. Eating and drinking at zero g were not troublesome when squeeze bottles and tubes were used, and urination presented no real difficulty. Some subjects suffered nausea, disorientation, loss of coordination, and other disturbances, but the majority reported that after they adjusted to the condition they found it "pleasant" and had a feeling of "well-being."21 As early as 1955, Simons concluded that weightlessness produced no abnormalities with regard to heart rate and arterial and venous blood pressure, while Henry, Simons' colleague in the Aerobee animal experiments, prophesied, "In the skilled pilot weightlessness will probably have very little significance."22 And in 1959, about a year after Project Mercury got underway, Gerathewohl remarked that "the majority of flying personnel enjoy the exposure to the subgravity state in our controlled experiments. We have reason to believe that even longer periods of absolute weightlessness can be tolerated if the crew is properly conditioned and equipped."23
Multiple G
Another problem perplexing aeromedical experts as the era of space flight neared was the effect on the human body of the heavy acceleration and deceleration forces, called "g loads," building up during rocket-propelled flights into space at speeds far greater than those yet experienced by man. Many fighter pilots in the Second World War had suffered momentary pain and blurred vision during "redout," when blood pooled in the head and eyes during an outside loop, or "blackout," when the heart suddenly could not pump enough blood to the head region as an airplane pulled out of a steep dive. Acceleration of a vehicle into space and the deceleration accompanying its return to the atmosphere would subject a man to g loads several times the normal accelerative force of gravity. In other words, for parts of a space mission a man would come to "weigh" several times what he normally did on Earth; a severe strain would be imposed on his body organs.
At the Aeromedical Field Laboratory in New Mexico, Harald J. von Beckh, a physician who had immigrated from Germany by way of the Instituto Nacional de Medicina Aeronéutica in Buenos Aires, was especially concerned about the abilit of a space traveler to tolerate the high deceleration forces of atmospheric entry after several hours of weightlessness. In the last few months before such research ended at Holloman, von Beckh inquired into the relationship between zero g and the multiplication of g. He added a steep downward spiral to the level, weightless portion of the Keplerian trajectory in order to impose heavy g loads on a test subject immediately after a half minute or so of weightlessness. After a number of these parabolic-spiral flights, he reported pessimistically, "Alternation of weightlessness and acceleration results in a decrease of acceleration tolerance and of the efficiency of physiologic recovery mechanisms … Because there is a decreased acceleration tolerance," he warned, "every effort must be made to reduce G loads to a minimum."24
Throughout the 1950s a substantial number of aeromedical experts concerned themselves with acceleration-deceleration loads per se, not necessarily in connection with the gravity-free state. Research on g forces reached back for decades, to the primitive period of aviation medicine. The state of knowledge with regard to the physiology of acceleration-deceleration was still hazy and fluid in the early fifties, although for at least 25 years aviation physicians in Europe and the United States had been studying blackout, redout, impact forces, and other effects of high g in aircraft.25 The V-2 and Aerobee animal rocket shots also had added to research data on the problem. But until the X-15 was ready, researchers had about exhausted the airplane as a tool for studying g loads, and from 1952 to 1958 experimentation with animal-carrying rockets was suspended in the United States. Consequently American scientists had to turn to two devices on the ground - the rocket-powered impact sled, used for studying the immediate onset of g loads, and the centrifuge, where the slower buildup of g could be simulated - to enlarge what they knew about the limits of human endurance of heavy acceleration and deceleration.
On December 10, 1954, Lieutenant Colonel John P. Stapp of the Aeromedical Field Laboratory gave an amazing demonstration of a man's ability to withstand immediate impact forces. Stapp rode a rocket-driven impact sled on the 3,550-foot Holloman research track to a velocity of 937 feet per second and received an impact force of 35 to 40 g for a fraction of a second as the sled slammed to a halt in a water trough.26 In February 1957 a chimpanzee rocketed down the track, now 5,000 feet long, braked to a stop, and survived a load of some 247 g for a millisecond, with a rate of onset of 16,000 g per second. And 15 months later, on the 120-foot "daisy track" at Holloman, Captain Eli L. Beeding, seated upright and facing backward, experienced the highest deceleration peak yet recorded on a human being - 83 g for .04 of a second, with 3,826 g per second as the calculated rate of onset. Afterward Beeding, recovering from shock and various minor injuries, judged that 83 g represented about the limit of human tolerance for deceleration.27
Such studies of deceleration were not directed primarily toward space missions but rather toward the problem of survival after ejection from or crashes in high-performance aircraft. The Holloman sled runs of the fifties, however, did broaden considerably the available data on the absolute limits of man's ability to endure multiples of g. And, perhaps more important, the New Mexico experiments in biodynamics were directly applicable to the problem of high g forces resulting from the uncushioned impact of a spacecraft on water or land. Stapp reasoned that a properly restrained, aft-facing human being could withstand a land impact of some 80 knots (135 feet per second) in a spacecraft if the g forces were applied transversely, or through the body, and if the spacecraft did not collapse on him.28
The centrifuge, the other laboratory tool used by students of acceleration-deceleration patterns, became increasingly useful in the fifties. The basic feature of the centrifuge was a large mechanical arm with a man-carrying gondola or platform mounted on the end, within which a test subject would be rotated at high angular velocities. Centrifuge experiments had more immediate pertinence to space medicine than impact sled tests, because on the "wheel" investigators could duplicate the relatively gradual buildup of g forces encountered during the launch and reentry portions of ballistic, orbital, or interplanetary flight. In the fifties, centrifuges existed at several places in the United States. The best-known and most used were at the Navy's Aviation Medical Acceleration Laboratory, Johnsville, Pennsylvania, and at the Aeromedical Laboratory at Wright-Patterson Air Force Base. During the decade, researchers at Johnsville, Wright-Patterson, and elsewhere simulated a wide variety of acceleration and deceleration profiles, using an almost equally wide variety of body positions and support systems, to compile an impressive quantity of data on the reactions of potential space pilots to heavy g forces.29
Just after the Second World War, Otto Gauer and Heinz Haber, who had conducted centrifuge experiments for the German Air Force, proposed a series of acceleration patterns, ranging from 3 g for 9½ minutes to 10 g for 2 minutes all of which would be tolerable for a space pilot.30 Then, in 1952, E. R. Ballinger, leader of the research program at Wright-Patterson, conducted one of the earliest series of centrifuge tests directed expressly toward the problem of g forces in space flight. Ballinger found that 3 g applied transversely would be the ideal takeoff pattern from the physiological standpoint, but he realized that the rocket burning time and velocity for such a pattern would be insufficient to propel a spacecraft out of the atmosphere. Consequently he and his associates subjected men to gradually increasing g loads, building to peaks of 10 g for something over two minutes. Chest pain, shortness of breath, and occasional loss of consciousness were the symptoms of those subjected to the higher g loads. The tests led Ballinger to the conclusion that 8 g represented the acceleration safety limit for a space passenger.31
Data gained from the first Soviet and American instrumented satellites of late 1957 and early 1958 showed that the atmosphere reached considerably farther out than scientists previously had realized. Until these disclosures aeromedical experts had assumed that the deceleration, or backward acceleration, forces of reentry, producing what was graphically described as an "eyeballs out" sensation, would be much greater than the acceleration during the ascent, or "eyeballs in," phase of the mission. Proceeding on this assumption, a team of physiologists from the Army, Navy, and Air Force had used the 50-foot centrifuge at the Navy's Johnsville installation to study the anticipated high reentry g buildup, exposing five chimpanzees to a peak of 40 g for one minute. Post-run examinations of the primates showed internal injuries, including heart malfunctions. It appeared that prolonged subjection to high g might be severely injurious or perhaps even fatal to a man.32
The tests conducted by Ballinger at Wright-Patterson and the interservice experiments with the chimpanzees on the Navy centrifuge featured frontward (eyeballs-in) application of g loads during the launch profile, backward application (eyeballs-out) during the reentry simulation, and the use of rather elaborate restraint straps and basic aircraft bucket seats as a support system. The problem of determining optimum body position and support was vigorously attacked by biodynamicists during 1957 and 1958. A series of especially careful studies on the Wright Air Development Center centrifuge indicated that when the subject was positioned so that the g forces were applied transversely and backward to the center of rotation, breathing became easier. Acceleration-deceleration patterns of 12 g for 4 seconds, 8 g for 41 seconds, and 5 g for 2 minutes were endured without great difficulty by practically all the volunteer subjects, some having even higher tolerance limits. Results of runs on the Johnsville centrifuge with the subjects in an aft-facing position for both acceleration and deceleration patterns also appeared favorable.33
The students of g forces tried various support devices in the late fifties in their search for ways to increase human tolerance to acceleration and deceleration loads. One specialist in the Wright-Patterson centrifuge group came up with a suit of interwoven nylon and cotton material, reinforced by nylon belting, and attached to the pilot seat at six places to absorb the g loads and distribute them more evenly over the entire body. Later, Wright-Patterson scientists using a nylon netting arrangement in conjunction with a contour couch were able to expose several men to a peak of 16.5 g for several seconds without any discoverable adverse effects. Other Air Force specialists experimented with subjects partially enclosed in a "rigid envelope," actually a plaster cast, as protection against both g-load buildup and impact forces. And von Beckh, whose concern with the weightlessness-deceleration puzzle led him to experiment with anti-g techniques, developed a device called "multi-directional g protection," a compartment that turned automatically to ensure that the g forces were always applied transversely on its occupant. Von Beckh's invention was used to protect a rat that went along on Beeding's record sled run in 1958, and a modified compartment carried three mice on a Thor-Able rocket launch the same year. Results in both experiments were encouraging.34
Navy scientists were especially interested in water immersion as a means of minimizing g loads. Researchers in Germany, Canada, and the United States had experimented with water-lined flying suits and submersion in water tanks, beginning in the 1930s. Specialists had carried out sporadic biodynamic tests with immersed rabbits and mice in the late forties at the Navy School of Aviation Medicine and, after the giant centrifuge began operation in 1952, in Johnsville.35
In 1956, R. Flanagan Gray, a physician at the Johnsville laboratory, designed an aluminum centrifuge capsule that could be filled with water and was large enough to hold a man. After some initial troubles installing the contraption on the centrifuge and perfecting an emergency automatic flushing mechanism, the "Iron Maiden," as it was rather inaccurately nicknamed, went into use. In March 1958, Gray, immersed to his ribs in a bathtub-like device developed at the Mayo Clinic during the Second World War, had endured 16 g of headward (head to feet) acceleration. Then, the next year, Gray enclosed himself in the Iron Maiden and, positioned backward to the center of rotation and immersed in water above the top of his head, held his breath during the 25-second pattern to withstand a peak of 31 g transverse acceleration for five seconds. This performance with the water-filled aluminum capsule established a new record for tolerance of centrifuge g loads.36
Nylon netting, multidirectional positioning, and water immersion were all promising methods for combating g forces and expanding human endurance limits. But netting had a troublesome tendency to bounce the subject forward as the g forces diminished, while directional positioning and water-immersion apparatus required more space and weight than would be available in a small, relatively light spacecraft.37 And considering the thrust limitations of the Thor, the Atlas, or the somewhat larger Titan ICBM, a small spacecraft was the only feasible design for an American manned satellite in 1958.
At the inception of the NASA manned satellite project, in the fall of 1958, the apparent solution to the problem of body support was an anti-g contrivance developed not by biodynamicists but by a group of practicing aerodynamicists in NACA's Pilotless Aircraft Research Division, part of the Langley Aeronautical Laboratory in Virginia. Maxime A. Faget, William M. Bland, Jr., Jack C. Heberlig, and a few other NACA engineers had designed an extremely strong and lightweight couch, made of fiber glass, which could be contoured to fit the body dimensions of a particular man. In the spring of 1958, technicians and shopmen at Langley molded the first of a series of test-model contour couches. The following July a group from Langley went to the Aviation Medical Acceleration Laboratory at Johnsville to try out their couch on the Navy's big centrifuge.38
The Navy biodynamicists and the NACA engineers experimented with the couch and various body positions in an effort to amplify a g-load tolerance. The couch made at Langley had been molded to fit the physical dimensions of Robert A. Champine, one of the foremost NACA test pilots. Champine rode the Johnsville centrifuge to a peak of 12 g on July 29, then departed for a conference on the Pacific Coast. The next day Navy Lieutenant Carter C. Collins volunteered to test the couch. Since his frame was smaller than Champine's, the Johnsville experts had to pack foam-rubber padding into the recesses of the fiber-glass bed. Collins then climbed into the centrifuge gondola and seated himself in the couch, the back angle of which was set forward 10 degrees. The 4,000-horsepower centrifuge motor whirled the gondola progressively faster. On the first run the loads reached a peak of 12 g. Five more runs pushed the peak to 18 g. Then, on the sixth try, using a grunting technique to avoid blackout and chest pains, Collins withstood a peak of 20.7 g, applied transversely for a duration of six seconds. Later that day, Gray, inventor of the Iron Maiden, rode the centrifuge with the contour couch and also endured a 20-g peak. The acceleration patterns to which Collins and Gray were exposed corresponded to a reentry angle of 7.5 degrees. At that time the optimum reentry angle being considered for a manned satellite, 1.5 degrees, theoretically would expose the spacecraft passenger to only 9 g.39
Mercury Couch
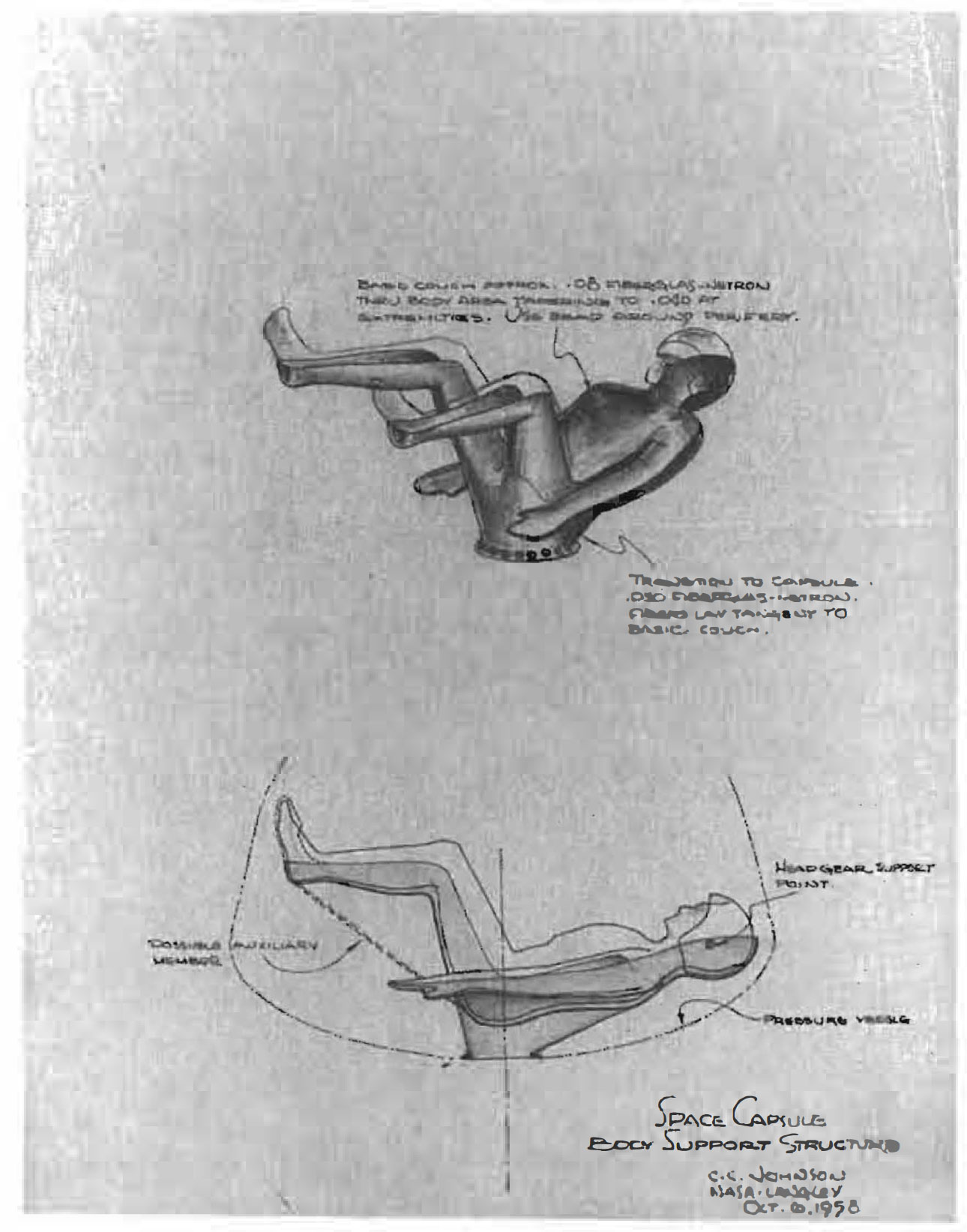
One of the most critical design and feasibility problems in the early days of Mercury was whether the astronaut could be safely restrained and supported through the succession of vibration levels, g forces, weightlessness, and more g forces that would occur in space flight. Langley laboratory engineers conceived the contour couch in 1958.
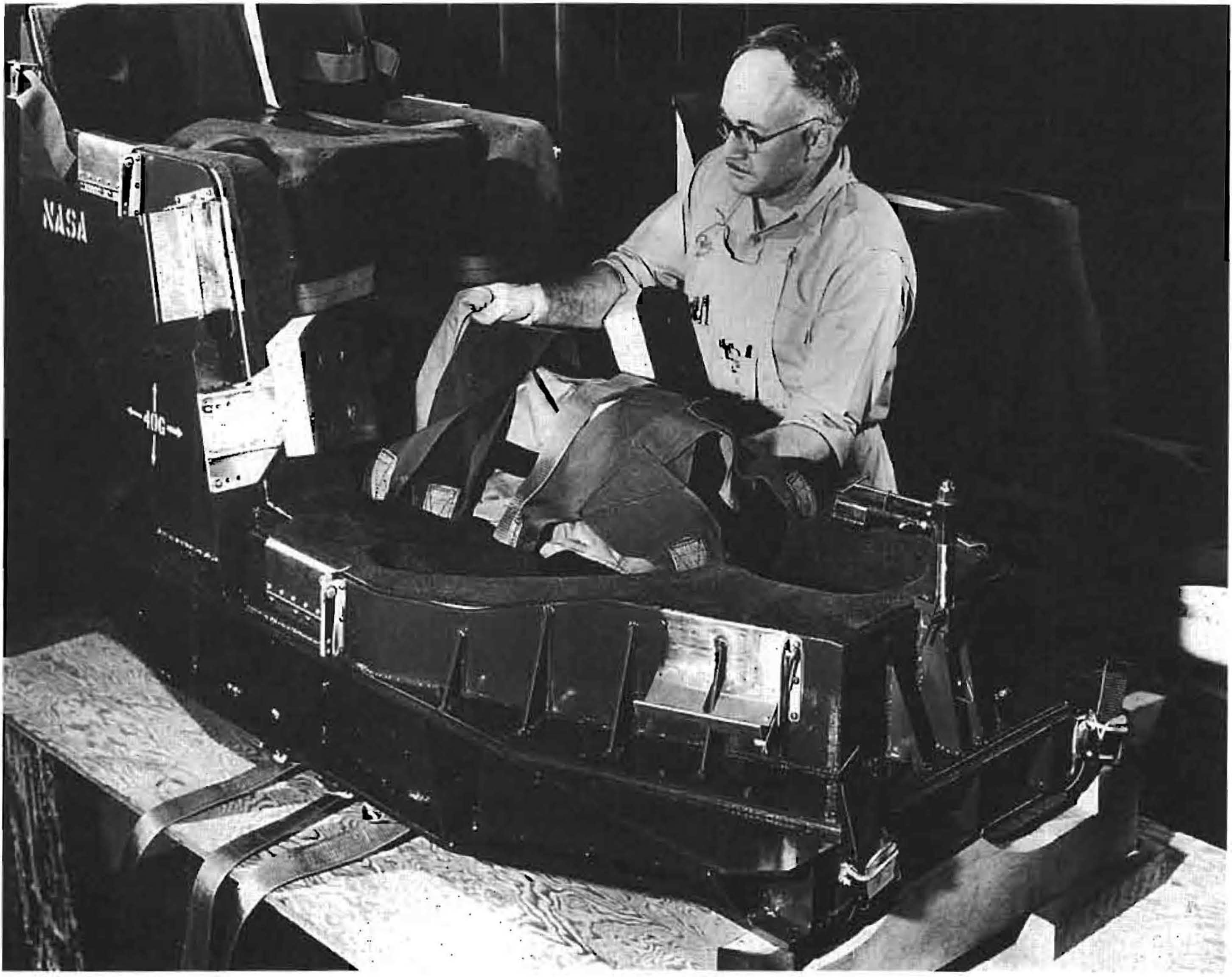
Refined it enough to try a model in 1959.
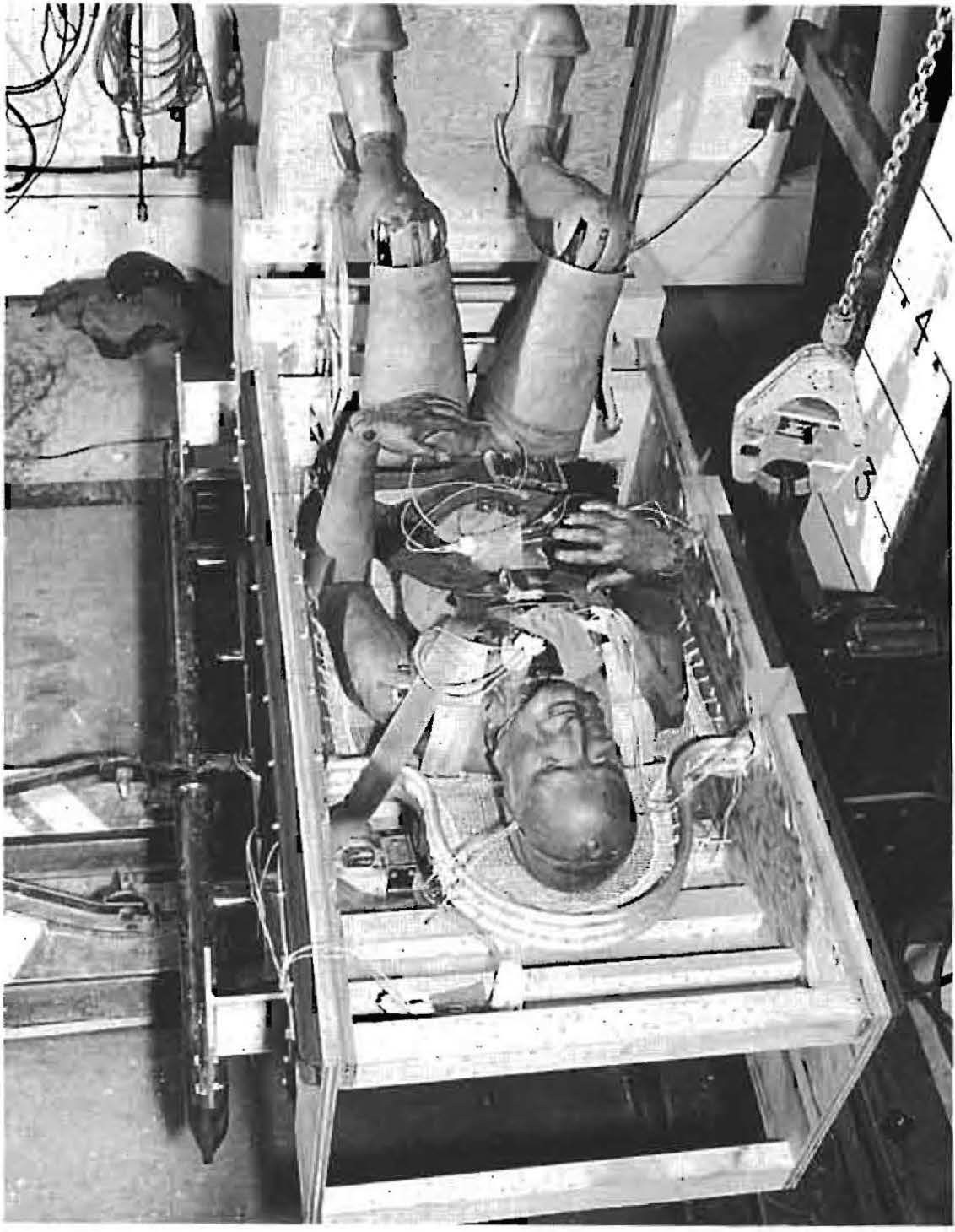
After the couch concept had been devised, there was the problem of a system to provide the contradictory combination of restraint, cushioning, and support. An early couch of nylon netting was ruled out because it bounced the occupant forward as g forces diminished.
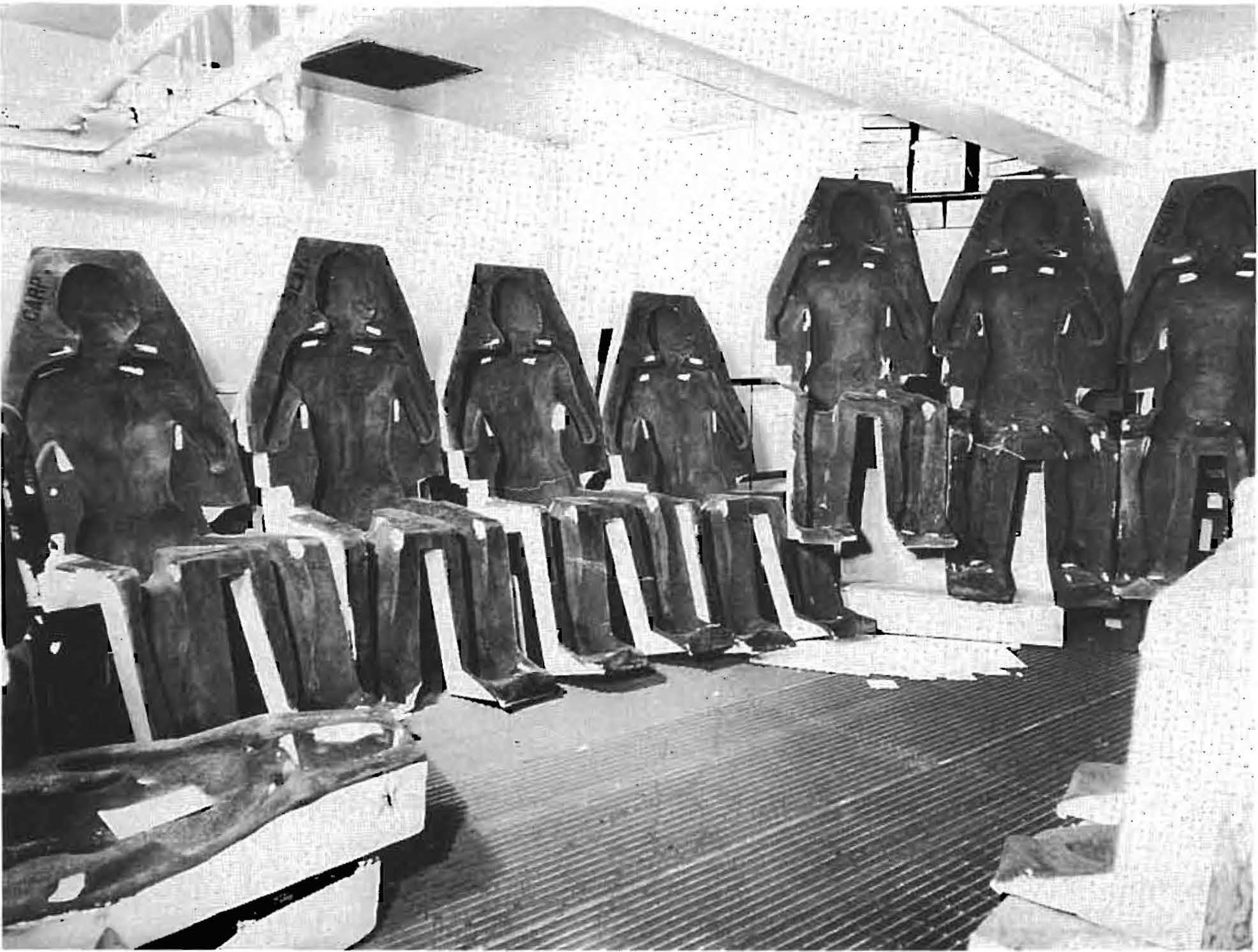
The final choice was fiber glass cast to the contour of each astronaut and equipped with restraining straps.
The NACA engineers, already working overtime on designs for a manned orbital capsule, were elated. It seemed that they finally had an effective anti-g device that was small enough and light enough to fit into a one-ton ballistic capsule they had in mind for the initial manned space venture.40 They had, in fact, made a major contribution to the protection of a space rider from sustained high g forces, although they did not fully realize as yet that body angles were more significant features of the couch than its contoured support.
The procedure ultimately used for protecting the Mercury astronauts from the g loads of acceleration to orbital velocity and deceleration during reentry represented a combination of the advantages gained from many experiments by military and other specialists in flight physiology, as well as from the ingenuity of the aeronautical engineers in NACA and NASA. Although the idea of using a hammock either for the basic support or in combination with the contour couch was perennially attractive to the human-factors experts in Project Mercury, all Mercury astronauts sat in essentially the same couch designed by Faget and his coworkers in the spring of 1958. But added to this basic technique were restraining straps, a semi-supine posture, frontward application of acceleration loads, and the reversal of the spacecraft attitude during orbit to permit frontward imposition of reentry loads as well. The final elements in the NACA-NASA campaign to minimize the effects of insertion-reentry g buildups was the use as astronauts of experienced test pilots provided by the military services. During the centrifuge experiments of the fifties such men had consistently proved capable of withstanding higher g forces than nonpilots.
Environmental Control
High-altitude atmospheric flight had necessitated much work related to two serious physiological problems of space flight - air supply and the pressure required for breathing in space. Research on these problems in the United States stretched back to 1918, when the Army began operation of a decompression chamber at Hazelhurst Field, Long Island. In the early 1930s the civilian aviator Wiley Post wore a pressure suit, looking like a deep-sea diver's outfit, for high-altitude flying. By the early fifties the typical jet pilot breathed pure oxygen for hours in an artificially pressurized cabin while wearing a pressurized flying suit as an extra protection in case of cabin decompression.41
Air compression, however, is not practicable above 80,000 feet. Travel outside the breathable atmosphere, whether into space or to the bottom of the sea, necessitates living inside a hermetically sealed compartment, a completely airtight ecological system in which carbon dioxide exhaled by the traveler is constantly replaced by an onboard supply of pure oxygen or some combination approximating the nitrogen-oxygen composition at sea level. In this area of space flight research - space cabin environment - the Air Force achieved preeminence in the early fifties with the development of the first sealed space cabin.
The sealed space cabin had two essential precursors. One was the sealed gondola for stratospheric ballooning, used by the Swiss twins Auguste and Jean Piccard in several flights to altitudes of around 10 miles in the 1930s and in the Explorer II ascent of 1935, which carried Army experimenters Orvil A. Anderson and A. W. Stevens to 72,335 feet and set a record that stood for 20 years.42 The other was the closed underwater environment of the bathysphere, used for many years in deep-sea exploration, and of the submarine. In the fifties, Air Force research on the sealed space cabin paralleled similar work by Navy scientists on an environmental control system for the new atomic-powered submarines, which were being designed to remain totally submerged for months.43
In 1952, Fritz Haber, of the Air Force School of Aviation Medicine, drew blueprints for a sealed chamber to be used for space medicine research; at the urging of Hubertus Strughold the Air Force let a contract for its construction. The Guardite Company of Chicago delivered a completed cabin in the summer of 1954.44 "Nobody took notice of a 'sealed cabin,'" recalled Strughold. "We had to have a name that would attract attention to our work. So I named it the 'Space Cabin Simulator.'"45
The cabin provided about 100 cubic feet of living space, room enough for an ordinary aircraft seat and a panel of lights, switches, and displays to test the psychological reactions of the subject. It had systems for air conditioning, oxygen supply and carbon dioxide absorption, urine distillation, and the recycling of the distilled urine together with air moisture to provide water pure enough to drink. Cabin pressure was maintained constantly at a level equivalent to an altitude of 18,000 to 25,000 feet.46
The space cabin simulator received its first national publicity in March 1956, when Airman D. F. Smith spent 24 hours in the chamber at San Antonio, performing a number of tasks for psychological monitoring and wearing instrumentation to record his heart action, temperature, and respiration rate. During the next two years, Lieutenant Colonel George R. Steinkamp, Captain Julian Ward, and George T. Hauty, who had charge of the simulations, gradually increased the duration of the tests. On February 16, 1958, four and a half months after Sputnik I and after seven days in the sealed chamber, Airman Donald F. Farrell stepped out to be greeted by a crowd of newsmen and by Senator Lyndon B. Johnson. In this, the most famous experiment ever run in the original space cabin simulator, Farrell had spent his week completely isolated in an environment that duplicated life inside a spacecraft in every respect except the weightless condition.47
The Farrell experiment provided no unexpected physiological data. But Hauty, chiefly interested in the psychological portion of the simulation, reported that the daily log kept by Farrell showed a deterioration from good spirits to "the seemingly abrupt onset of frank hostility." Farrell's mental condition "reached the point of becoming the single conceivable reason for a premature termination of the flight." Hauty noted that Farrell's proficiency at tasks assigned to him also deteriorated severely as the experiment progressed.48
The psychological data from the early space cabin simulator tests, as well as observation of subjects in the isolation chamber at Wright-Patterson Air Force Base, were not encouraging. Major Charles A. Berry, an Air Force physician who later would work closely with the astronauts in Project Mercury, perhaps expressed the consensus among space medicine investigators by 1958: "The psychological problems presented by the exposure of man to an isolated, uncomfortable void seem to be more formidable than the physiological problems."49
Matter From Space
Even after enclosing himself in a sealed cabin and adjusting to prolonged isolation, the first man in space ran the danger of being killed by decompression if his cabin were punctured by one of the myriad meteoroids, ranging in size from less than a millimeter up to several meters, that constantly bombard Earth's atmosphere.50 Impact with a meteoroid, even one the size of a BB shot, conceivably could put a hole in the structure of a spacecraft and cause death to its occupant through either gradual or explosive cabin decompression.
In the forties and early fifties scientists varied widely in their guesses as to the probability of meteoroid impact. Fletcher G. Watson, a Harvard University astronomer, predicted in 1946 that at least one of every 25 space ships going to the Moon would be destroyed by collision with a meteoroid. Two years later George Grimminger, a mathematician with the Rand Corporation, estimated that a spacecraft with an exposed area of 1,000 square feet would be hit by a particle with a diameter of ½ millimeter only about once every 15 years. As late as 1951, however, Fred L. Whipple of Harvard, one of the principal American authorities on meteoroids, was rather pessimistic about the chances of avoiding meteoroid penetration and suggested thick shielding on the spacecraft to guard against structural damage.51
The early instrumented satellites sent up by the Soviet Union and the United States did much to dispel the fears of the space flight enthusiasts about meteoroids. The American satellite Explorer I, launched in January 1958, recorded only seven hits by micrometeoroids - particles considerably less than a millimeter in diameter - during the first month of its orbital life. Apparently none of these pieces of matter penetrated the satellite's outer skin. Data from the much larger Russian Sputnik III, sent into orbit in May 1958, indicated that an orbiting spacecraft with a surface of 1,000 square meters (10,760 square feet) would be hit by a meteoroid weighing at least one gram only once every 14,000 hours. And Explorer VI, orbited by the United States in the late summer of 1959, encountered meteoroid dust particles only 28 times during the first two days it was in orbit.52 These data prompted a human-factors specialist for one of the major aerospace firms to conclude that for low orbital missions in a manned spacecraft "the danger from meteorite [sic] penetration is minor to negligible in comparison to the other hazards of such flights."53 Nevertheless, Project Mercury astronauts would wear a full-pressure suit, a closed ecological system in itself, so that if cabin decompression occurred each astronaut could live until his space capsule could be brought back to Earth.
Space Radiation
In addition to weightlessness, g loads, air, water, and food supply, isolation, and meteoroids, the problems of space flight included protecting the passenger from different kinds of electromagnetic radiation found above the atmosphere. Of the varieties of radiations in space the most mysterious is cosmic radiation, the source of which presents one of the grandest puzzles in nuclear astrophysics. Some of this radiation possibly comes from the Sun, but the preponderance of the cosmic rays bombarding Earth's atmosphere evidently originates outside the solar system - thus the term "cosmic" radiation. High-energy cosmic ray primaries - subatomic particles, of which about 90 percent are protons of hydrogen and helium - slam into the atmosphere at velocities approaching the speed of light. Fifteen to 25 miles above Earth, the cosmic ray primaries collide with atoms and molecules in the thickening atmosphere, are broken up, and are converted into lower-energy rays called secondaries. Above 25 miles the atmosphere becomes too thin to absorb the cosmic ray primaries; since they are capable of penetrating a thick lead wall, it was futile to try to shield a spacecraft pilot completely. So in the early 1950s medical researchers, assuming that a space pilot would be exposed to some cosmic radiation, approached the problem primarily from the angle of establishing how large a dose a human being could tolerate.54
As with weightlessness and g-load research, the best postwar device for studying cosmic radiation was the instrumented sounding rocket. But the last of the rocket experiments with primates occurred in May 1952. From that time until animal rocket shots resumed in 1958, the only upper-atmospheric research rockets fired in the country were occasional Aerobees, launched by the Air Force to altitudes of about 150 miles.55 These shots, carrying only instruments, brought back a modicum of data on cosmic rays. The prime instrument for cosmic ray research from 1952 to 1958 was the oldest vehicle for human flight, the balloon. The postwar development of sturdier, larger, polyethylene balloons to replace rubber aerostats made possible higher and higher ascents with increasingly heavier loads. At the same time the expansion of balloon technology, leading to an increasing number of giant, shiny spheres floating over the United States, multiplied reports of and popular interest in "Unidentified Flying Objects."56
In the balloon-borne space radiation experiments of the fifties, the Navy carried out some notable manned ascents into the stratosphere. On November 8, 1956, for example, Lieutenant Commanders Malcolm D. Ross and M. L. Lewis, as part of the Navy's Strato-Lab program of manned ascents from northern latitudes, reached 76,000 feet, then an altitude record. Less than a year later Ross and Lewis sat in their cramped sealed gondola as their huge polyethylene balloon ascended to nearly 86,000 feet. And in late June 1958 the same two Navy aerostation veterans remained in the 70,000-80,000-foot region for almost 35 hours.57
The Navy also pioneered in the use of balloon-launched rockets (rockoons). The first successful rockoon launch occurred in August 1952 when, from a ship off the coast of Greenland, a University of Iowa team headed by physicist James A. Van Allen sent up a balloon from which a rocket ignited at 70,000 feet and climbed to an altitude of nearly 40 miles. The Navy did most of its upper-atmospheric research, however, with instrumented balloon flights carrying small organisms and insects. In May 1954, for example, General Mills, Incorporated, under contract to the Office of Naval Research, launched a polyethylene balloon, with a capacity of 3 million cubic feet, that carried cosmic ray emulsions - plates designed for recording the tracks of ionizing particles - to an altitude of 115,000 feet. Five years later, from Sioux Falls, South Dakota, Raven Industries launched an Office of Naval Research balloon biological package to a record altitude of 148,000 feet.58
The center of Air Force balloon research in the early 1950s was the Aeromedical Field Laboratory in New Mexico. From July 21, 1950, when Air Force personnel launched the first polyethylene balloon at Holloman Air Force Base, to December 18, 1958, the scientists at the field laboratory sent up 1000 research balloons, although only a small number of these ascents were designed expressly for cosmic ray study. In 1953 the Holloman researchers moved most of their balloon experiments to the northern United States, in the higher geomagnetic latitudes, where they could obtain increased exposure to cosmic ray primaries. During the next year they sent aloft a collection of radish seeds on a series of flights, compiling some 251 hours of exposure of the seeds above 80,000 feet. Monkeys, mice, rats, hamsters, and rabbits also drifted upward in balloons launched by Winzen Research, Incorporated, as a Holloman contractor, from Sault Ste. Marie, Michigan. The most interesting effect observed among the various test subjects was a striking increase in the number of gray hairs on black mice exposed to the high altitudes.59
The first solo manned ascent into the stratosphere was also principally an undertaking of the field laboratory at Holloman. In 1956 field laboratory experimenters inaugurated Project Manhigh, a series of flights from northern sites using Winzen balloons, to test man's ability to live for prolonged periods in a sealed-cabin environment like that inside a spacecraft and to gather new data on cosmic radiation. David Simons, head of the Space Biology Branch at Holloman, was project officer for the Manhigh ascents. The initial flight, from Fleming Field, Minnesota, took place on June 2, 1957. Captain Joseph W. Kittinger stayed aloft inside his sealed gondola for nearly seven hours, breathing pure oxygen, making visual observations, and talking frequently with John P. Stapp, the flight surgeon, and other physicians on the ground. Kittinger spent two hours above 92,000 feet; his maximum altitude during the flight was 96,000 feet.60
About nine weeks later Simons himself entered the space equivalent region, suspended in a sealed capsule below a 3-million-cubic-foot polyethylene balloon launched from an open-face mine near Crosby, Minnesota. Simons exceeded Kittinger's mark for both duration and altitude, staying aloft 32 hours and remaining at 101,000 feet for about 5 hours. Simons was the first man in history to see the Sun set and then rise again from the edge of space. In the Manhigh I gondola he spent more time than anyone before him looking upward at the blackness of space and outward at the white and blue layers of the atmosphere. "The capsule seemed like a welcome window permitting a fabulous view and precious opportunities, not a prison or an enclosure," he related after the flight.61
In October 1958 an excessive temperature rise in the capsule forced a premature termination of the third Manhigh flight, carrying Lieutenant Clifton M. McClure.62 Yet McClure's ascent, together with those of Kittinger and Simons, proved the workability of the sealed cabin for sustaining human life where "the environment is as hostile and very nearly as different in appearance as one would expect to observe from a satellite."63 The environmental control system of the Manhigh capsule and the instrumentation for physiological telemetering were strikingly similar to those later used in the Mercury spacecraft.
With regard to cosmic radiation, however, the Manhigh flights, like numerous rocket, balloon, and laboratory experiments of previous and succeeding years, returned data that were either negative or inconclusive. During the Manhigh II ascent two containers of bread mold were attached to the underside of the capsule, and Simons wore emulsion plates on his arms and chest to measure cosmic ray penetration. The plates did show indications of several hits by so-called "heavy" primaries - cosmic ray particles made up of nuclear particles heavier than are found in hydrogen or helium - but years later the skin in the area of the plates revealed no effects of radiation.64
All these experiments left most scientists as reluctant to speculate about the hazards from cosmic rays in flight as they had been in the early fifties. Simons felt that in manned orbital flights following roughly equatorial orbits, where the spacecraft remained within the protective shielding of Earth's magnetic fields, the spacecraft pilot would be in no danger from cosmic radiation. Yet he remained troubled by the possibility that a solar flare, a sudden burst of energy from the Sun, might precipitate a great increase in cosmic ray intensity during a space mission. About a twentyfold multiplication of cosmic radiation accompanied a solar flare of February 1956. Simons' concern with solar flares led him to the conclusion that continuous voice contact between ground stations and the space pilot would be essential, as well as stepped-up efforts to predict the flares.65
All proponents of manned space flight were alarmed when information transmitted from the first three Explorer satellites, launched during the first half of 1958, disclosed the existence of a huge envelope of radiation beyond the ionosphere. Evidently consisting of protons and electrons trapped in Earth's magnetic field, the radiation layer begins about 400 miles out in space and doubles in intensity about every 60 miles before tapering away about 1200 miles from Earth. This discovery was the first "Van Allen belt," named after James A. Van Allen, United States director of the International Geophysical Year radiation experiments. The Pioneer III probe, launched in December 1958, failed to reach escape velocity, but it did reveal that the radiation zone consisted not of one belt but of two at least - an inner belt of high-energy particles and an outer belt of less energetic particles. Two earlier Pioneer shots, in October and November, had shown that while the radiation zone was several thousand miles deep, it did not extend into space indefinitely.66 Quite obviously, the doughnut-shaped Van Allen belts would pose a serious threat for manned travel in high orbits or interplanetary voyages. In the early manned ventures into space, however, a spacecraft could be placed in an orbit 100 to 150 miles from Earth, high enough to be free of atmospheric frictional drag, yet low enough to stay under the Van Allen radiation.67
The radiation hazards of space flight also include solar radiation. Solar heat, ultraviolet rays, and x-rays all become much more intense beyond the diffusive atmosphere of Earth, but they can be adequately counteracted by space cabin insulation, shielding, refractive paint, and other techniques. Advanced space missions may subject astronauts to dangers from other kinds of radiation, such as the radiation belts surrounding other planets or the radioactivity produced by a spacecraft with a nuclear powerplant.68
A Reason for Research
During 1958, scientists and engineers, both military and civilian, talked more openly than they had in previous years about radiation dosages, meteoroid penetration, weightlessness, and the other anomalies of space travel. They received a considerably more respectful hearing. What made members of the Congress and Americans in general responsive to such discussions and interested in past research and future plans for space exploration were the ever-larger scientific satellites launched by the Soviet Union, beginning October 4, 1957. In the midst of the nationalistic humiliation following the Sputniks, not only space rocketry but also medical research with rockets received an invaluable boost. In May 1958, Air Force physicians sent mice along on three reentry tests of the Able ablation nose cone for the Thor. Then, the following December and in May 1959, the Navy School of Aviation Medicine dispatched monkeys, sea-urchin eggs and sperm, molds, tissues, and seeds on two test firings of the Jupiter intermediate range missile, carried out by the Army Ballistic Missile Agency.69
The new focus on space, the new curiosity about what went on beyond the atmosphere, the determination to "catch up" in the space race - these sentiments redounded to the benefit of those Americans who had been trying to solve the biological and technological puzzles of manned space flight long before there was a space race. Their principal stimulus was not international prestige or the drive for technological supremacy; it was a desire to discover the undiscovered, to probe into the unknown. And they believed that wherever man's instruments went, man should follow. The proponents of manned space flight in the United States could be found in several locations - in the military, in some universities, in the aerospace industry, even in the Congress. But an especially zealous contingent worked for NACA. Ultimately its members would become the engineering and managerial nucleus of the American program to rocket a man into orbit around Earth and bring him back.
- On advances in telemetry since the Second World War, see Wilfred J. Mayo-Wells, "The Origins of Space Telemetry," in Eugene M. Emme, ed., The History of Rocket Technology: Essays on Research, Development, and Utility (Detroit, 1964), 253-268; also published in Technology and Culture, IV (Fall 1963), 499-514.X
- For the German work in aeromedicine during the 1930s and early 1940s, see U.S. Air Force, German Aviation Medicine, World War II (2 vols., Washington, 1950).X
- Harry G. Armstrong, Principles and Practices of Aviation Medicine (3 ed., Baltimore, 1952). Armstrong later became a major general.X
- Harry G. Armstrong, Heinz Haber, and Hubertus Strughold, "Aero Medical Problems of Space Travel, Panel Meeting, School of Aviation Medicine," Journal of Aviation Medicine, XIX (Dec. 1949), 383-417; Hubertus Strughold, interview, San Antonio, April 24, 1964. People interested in the physiology and psychology of extra-atmospheric flight have devised a number of terms to describe their field of investigation - biomedicine, space biology, astrobiology, bioastronautics, aerospace medicine. The most suitable single term seems to be that used by Strughold, "space medicine." It is used throughout this work except where more precise terminology, such as "biodynamics," appears appropriate.X
- Shirley Thomas, Men of Space (6 vols., Philadelphia, 1960-1963), IV, 234-250; USAF Air Training Command, History of the United States Air Force, Pamphlet 190-1, Randolph Air Force Base, Tex., 1961, 19; Strughold interview. Siegfried J. Gerathewohl, a psychologist, and Hans-Georg Clamann, a physiologist, remained with the School of Aviation Medicine but did not become members of the Department of Space Medicine.X
- Clayton S. White and Otis O. Benson, eds., Physics and Medicine of the Upper Atmosphere: A Study of the Aeropause (Albuquerque, 1952).X
- Hubertus Strughold, "Basic Environmental Problems Relating Man and the Highest Regions of the Atmosphere as Seen by the Biologist," ibid., 32. On the concept of space equivalence see also Strughold, Heinz Haber, Konrad Buettner, and Fritz Haber, "Where Does Space Begin? Functional Concept of the Boundaries between the Atmosphere and Space," Journal of Aviation Medicine, XXII (Oct., 1951), 342-357; Strughold, "Atmospheric Space Equivalence," Journal of Aviation Medicine, XXV (Aug., 1954), 420-424; Strughold, "The Medical Problems of Space Flight," International Record of Medicine, CLXVIII (1955), 570-575; and Strughold, "A Simple Classification of the Present and Future Stages of Manned Flight," Journal of Aviation Medicine, XXVII (Aug., 1956), 328-331.X
- "Thirty-Five Years of Winged Rocket Flight," Thiokol Magazine, II (1963), 10; Hubertus Strughold, "Introduction," to Morton Alperin, M. Stern, and H. Wooster, eds., Vistas in Astronautics: First Annual Astronautics Symposium (London, 1958), 283.X
- See Burt Rowen, "Human Factors Support of the X-15 Program," in Kenneth F. Gantz, ed., Man in Space: The United States Air Force Program for Developing the Spacecraft Crews (New York, 1959), 216-221; Stanley C. White, "Progress in Space Medicine," paper, Second World and Fourth European Aviation and Space Medicine Congress, Rome, Oct. 27-31, 1959; and Richard E. Day, "Training Aspects of the X-15 Program," in The Training of Astronauts (Washington, 1961), 5-14.X
- Otto Gauer and Heinz Haber, "Man under Gravity-Free Conditions," in German Aviation Medicine, World War II, I, 641-643.X
- David Bushnell, "History of Research in Subgravity and Zero-G at the Air Force Missile Development Center, 1948-1958," Air Force Missile Development Center, 1959, 3-7; David G. Simons, "Use of V-2 Rocket to Convey Primate to Upper Atmosphere," Air Force Tech. Report 5821, Air Materiel Command, Wright-Patterson Air Force Base, Ohio, May 1959; James P. Henry et al., "Animal Studies of the Subgravity State During Rocket Flight," Journal of Aviation Medicine, XXIII (Oct. 1952), 421-423; David G. Simons, interview, San Antonio, April 24, 1964. A breezy popular account of the Holloman shots with V-2s and Aerobees is Lloyd Mallan, Men, Rockets, and Space Rats (Rev. ed., New York, 1962), 84-116.X
- Andrei G. Kousnetzov, "Some Results of Biological Experiments in Rockets and Sputnik II," Journal of Aviation Medicine, XXIX (Nov. 1958), 781-784; S. M. Polovskov and B. A. Mirtov, "Study of the Upper Atmosphere by Means of Rockets at the U.S.S.R. Academy of Sciences," and A. V. Pokrovskii, "Study of the Vital Activity of Animals during Rocket Flights in the Upper Atmosphere," in F. J. Kreiger, ed., A Casebook on Soviet Astronautics (2 vols., Santa Monica, Calif., 1956-1959), II, 151-172; Siegfried J. Gerathewohl, Principles of Bioastronautics (Englewood Cliffs, N.J., 1964), 91-95.X
- Harald J. von Beckh, "Human Reactions during Flight to Acceleration Preceded by or Followed by Weightlessness," Aerospace Medicine, XXX (June 1959), 391.X
- Fritz Haber and Heinz Haber, "Possible Methods of Producing the Gravity-Free State for Medical Research," Journal of Aviation Medicine, XXI (Oct. 1950), 395-400.X
- Allen C. Fisher, "Aviation Medicine on the Threshold of Space," National Geographic, CVI (Aug. 1955), 257; Siegfried J. Gerathewohl, "Weightlessness," in Gantz, ed., Man in Space, 115.X
- Ibid.X
- Bushnell, "Research in Subgravity and Zero-G at the Air Force Missile Development Center," 12-17; James H. Hanrahan and David Bushnell, Space Biology: The Human Factors in Space Flight (New York, 1960), 137, 139-140; Green Peyton and Jean Evans, "History, Aerospace Medical Division, Air Force Systems Command: Reorganization, 1 November 1961-30 June 1962," Hist. Publication 62-180, Brooks Air Force Base, Tex., 1962, 93-97.X
- Hanrahan and Bushnell, Space Biology, 139-140; "USAF School Simulates Living in Space," Aviation Week, LXVIII (Jan. 27, 1958), 49-51; Siegfried J. Gerathewohl, Oskar L. Ritter, and Herbert D. Stallings, "Producing the Weightless State in Jet Aircraft," Report 57-143, Air Force School of Aviation Medicine, Aug. 1957.X
- Gerathewohl, Principles of Bioastronautics, 211-234; Siegfried J. Gerathewohl and Herbert D. Stallings, "Experiments during Weightlessness: A Study of the Oculo-Agravic Illusion," Journal of Aviation Medicine, XXIX (July 1958), 504-515; Julian E. Ward, Willard R. Hawkins, and Herbert D. Stallings, "Physiologic Response to Subgravity I: Mechanics of Nourishment and Deglutition of Solids and Liquids," Journal of Aviation Medicine, XXX (March 1959), 151-154, and "Physiologic Response to Subgravity II: Initiation of Micturition," Aerospace Medicine, XXX (Aug. 1959), 572-575.X
- "They Float through the Air," Astronautics, IV (Feb. 1959), 42; Hanrahan and Bushnell, Space Biology, 147-148; William Leavitt, "The Weird World of Weightlessness," Air Force, XLII (April 1959), 113.X
- Gerathewohl, "Weightlessness," 108-132 . The Navy School of Aviation Medicine experiments also employed parabolic aircraft flights, while the Lewis studies featured use of a tall drop-tower.X
- David G. Simons, "Review of Biological Effects of Subgravity and Weightlessness," Jet Propulsion, XXV (May 1955), 209-211; Fisher, "Aviation Medicine on the Threshold of Space," 257.X
- Gerathewohl, "Weightlessness," 132. See also Oskar L. Ritter and Siegfried J. Gerathewohl, "The Concepts of Weight and Stress in Human Flight," paper no. 58-154, Air Force School of Aviation Medicine, June 26, 1958.X
- Von Beckh, "Human Reactions during Flight to Acceleration Preceded by or Followed by Weightlessness," 391-406. After the termination of zero-g flights at Holloman and Randolph, weightless experiments in aircraft continued at Wright-Patterson and at the Air Force Flight Test Center, Edwards Air Force Base, Calif.X
- See Mae M. Link and Hubert A. Coleman, Medical Support of the Army Air Forces in World War II (Washington, 1955).X
- Hanrahan and Bushnell, Space Biology, 86-88; David Bushnell, "Major Achievements in Biodynamics: Escape Physiology at the Air Force Missile Development Center, 1953-1958," Air Force Missile Development Center, 1959, 10-13; Martin and Grace Caidin, Aviation and Space Medicine (New York, 1962), 199-203; Mallan, Men, Rockets, and Space Rats, 99-116. Stapp had taken his first rocket sled ride in 1947 at Edwards Air Force Base, Calif. He became Chief of the Aeromedical Field Laboratory in 1953 and made several more rides before his record run on Dec. 10, 1954. See John P. Stapp, "Tolerance to Abrupt Deceleration," in Collected Papers on Aviation Medicine, Presented at Aeromedical Panel Meetings of the Advisory Group for Aeronautical Research and Development, North American Treaty Organization (London, 1955), 122-169.X
- Eli L. Beeding, Jr., and John D. Mosely, "Human Deceleration Tests," Air Force Missile Development Center, Jan. 1960; Hanrahan and Bushnell, Space Biology, 93-94. The "daisy track" was named for a popular make of air rifle, because it was originally designed as a compressed-air catapult system. From 1955 to 1959 it used a cartridge system.X
- John P. Stapp, "Biodynamics of Space Flight," in Gantz, ed., Man in Space, 68.X
- See William J. White, A History of the Centrifuge in Aerospace Medicine (Santa Monica, Calif., 1964).X
- Gauer and Haber, "Man under Gravity-Free Conditions," 641-643.X
- Hanrahan and Bushnell, Space Biology, 72.X
- Ibid., 75-76. The largest centrifuge in operation in the United States during the 1950s was the Navy's mechanical arm at Johnsonville, with a radius of 50 feet and a capability of 40 g. The Johnsville facility had a device allowing the gondola to be gimbaled to simulate buffetings and cross-currents. The Wright Air Development Center centrifuge, on the other hand, had a radius of 20 feet and a capability of 20 g. Instead of a gondola it featured an open platform, which could be whirled in one plane only.X
- Ibid., 77; John P. Stapp, "Acceleration: How Great a Problem?" Astronautics, IV (Feb. 1959), 38-39, 98-100.X
- Ibid., 77, 105-109; memo for files, Gerard J. Pesman, Human Factors Br., Space Task Group, "Present Status - Major Systems, Pilot Support and Restraint" [about Feb. 1959]; Harald J. von Beckh, "Multidirectional G-Protection in Space Vehicles," Journal of the British Interplanetary Society, XVI (Sept.-Oct. 1958), 531.X
- Hanrahan and Bushnell, Space Biology, 96-98.X
- Carl C. Clark and James D. Hardy, "Preparing Man for Space Flight," Astronautics, IV (Feb. 1959), 18-21, 88; Clark and R. Flanagan Gray, "A Discussion of Restraint and Protection of the Human Experiencing the Smooth and Oscillating Accelerations of Proposed Space Vehicles," U.S. Naval Air Development Center, Dec. 29, 1959, 26-46. During 1957-1958 scientists at the Wright Air Development Center also carried out water-immersion studies, using a coffin-like container. Peak accelerations on the limited Wright centrifuge were only about 16 g, but the durations of the acceleration patterns were longer.X
- John P. Stapp, interview, San Antonio, April 24, 1964.X
- Maxime A. Faget, interview, Houston, Jan. 3, 1964, and Aug. 24, 1964; Gerard J. Pesman, interview, Houston, March 17, 1964; James M. Grimwood, Project Mercury: A Chronology, NASA SP-4001 (Washington, 1963), 20. For USAF concept of rotating couch, see p. 96.X
- Faget interview; Clark and Gray, "A Discussion of Restraint and Protection," 26; Pesman memo; John Dille, ed., We Seven, by the Astronauts Themselves (New York, 1962), 110-112.X
- Clark and Gray, "A Discussion of Restraint and Protection," 26; Pesman memo. Faget and his men became even happier in December 1958, during the early days of Project Mercury, when a second Langley couch, its back angle raised 8 degrees, supported Lt. Carter C. Collins on the Navy centrifuge during a peak of 25 g for approximately 10 seconds.X
- Stanley R. Mohler, "Wiley Post's Aerospace Achievements," Airpower Historian, XI (July 1964), 66-70; Frederick R. Ritzinger, Jr., and Ellis G. Aboud, "Pressure Suits - Their Evolution and Development," Air University Review, XVI (Jan.-Feb. 1965), 23-32.X
- Caidin and Caidin, Aviation and Space Medicine, 49-54; Eugene M. Emme, Aeronautics and Astronautics: An American Chronology of Science and Technology in the Exploration of Space, 1915-1960 (Washington, 1961), 26, 29, 30, 33, 160, 162; Auguste Piccard, Between Earth and Sky, trans. Claude Apcher (London, 1950), and Earth, Sky, and Sea, trans. Christina Stead (New York, 1956). For a useful survey of research in the physiology of high-altitude living, see From the Mountains to the Moon: Some Historical Aspects of Survival at Great Heights (undated microfilm, produced by Biomedical Laboratory, University of California at Los Angeles, NASA Historical Archives, Washington).X
- Hubertus Strughold, "The U.S. Air Force Experimental Sealed Cabin," Journal of Aviation Medicine, XXVII (Feb. 1956), 50; Hanrahan and Bushnell, Space Biology, 24-26.X
- Strughold, "Air Force Experimental Sealed Cabin," 50-51; Hans-Georg Clamann, interview, San Antonio, April 23, 1964.X
- Strughold interview.X
- "USAF School Simulates Living in Space," 49-55.X
- Emme, Aeronautics and Astronautics, 81, 95; Strughold interview; George R. Steinkamp and Willard R. Hawkins, "Medical Experimentation in a Sealed Cabin Simulator," in Otis O. Benson and Hubertus Strughold, eds., Physics and Medicine of the Atmosphere and Space (New York, 1960), 370-376.X
- George T. Hauty, "Human Performance in Space," in Gantz, ed., Man in Space, 84-108. Besides the aircraft weightless and sealed-cabin programs, the School of Aviation Medicine carried on various other research activities in space medicine. As early as 1947 scientists at the school were studying the ecological conditions on other planets. From this and other research came Strughold's The Green and Red Planet: A Physiological Study of the Possibility of Life on Mars (Albuquerque, 1953). Throughout the 1950s and to the present the Department of Space Medicine at the school has done research on the atmospheric composition of other planets, photosynthesis as a means of air supply, and other subjects.X
- Charles A. Berry, "The Environment of Space in Human Flight," Aeronautical Engineering Review, XVII (March 1958), 38; George E. Ruff, "Isolation," Astronautics, IV (Feb. 1959), 22-23, 110-111. After 1951 psychologists in several universities in the United States and Canada conducted sensory deprivation studies, laboratory experiments in which a subject was immersed in water in a blackened room and thus was deprived of his visual, auditory, and kinesthetic senses. The experiments indicated "a general loosening of the subject's ability to perceive reality and the weakening of the stable norms against which perception is evaluated." Later studies at the Navy Air Crew Equipment Laboratory in Philadelphia, the Air Force School of Medicine, and the Wright Air Development Center demonstrated, however, that stable, well-trained persons "can endure severe restrictions of their natural habitat for relatively long periods of time without significant degradation." Gerathewohl, Principles of Bioastronautics, 308, 311. See also D. Ewen Cameron et al., "Sensory Deprivation: Effects upon the Functioning Human in Space Systems," in Bernard E. Flaherty, ed., Psychophysiological Aspects of Space Flight (New York, 1961), 225-237.X
- Among astronomers, astrophysicists, and space flight experts there is considerable variation in the uses and definitions of the terms "meteoroid," "meteorite," and "meteor." Throughout this study the authors have employed what is apparently standard NASA terminology: a meteoroid is a solid object larger that a molecule and smaller than an asteroid, moving in interplanetary space; a meteorite is such an object that reaches Earth's surface without completely vaporizing in the atmosphere; and a meteor is the light phenomenon resulting from a meteoroid's entrance into the atmosphere.X
- Hanrahan and Bushnell, Space Biology, 31; George Grimminger, "Probability That a Meteorite Will Hit or Penetrate a Body Situated in the Vicinity of the Earth," Journal of Applied Physics, XIX (Oct. 1948), 947-956; Fred L. Whipple, "Meteoric Phenomena and Meteorites: The Conquest of Interplanetary Space," in White and Benson, eds., Physics and Medicine of the Upper Atmosphere, 137-170. On the meteoroid phenomenon see also Whipple, "Meteorite Material in Space," in Benson and Strughold, eds., Physics and Medicine of the Atmosphere and Space.X
- Hanrahan and Bushnell, Space Biology, 31.X
- A. B. Thompson, "Physiological and Psychological Considerations for Manned Space Flight," Report E9R-12349, Rev., Chance Vought Aircraft, Inc., Dallas, July 7, 1959, 115.X
- On cosmic radiation see, for example, James A. Van Allen, "The Nature and Intensity of the Cosmic Radiation," in White and Benson, eds., Physics and Medicine of the Upper Atmosphere, 239-266; Joseph A. Connor, "Space Radiation Protection," NASA-MSC fact sheet No. 106; Hermann J. Schaeffer, "Appraisal of Cosmic-Ray Hazards in Extra-Atmospheric Flight," in Alperin, Stern, and Wooster, eds., Vistas in Astronautics, 291-298; Gerathewohl, Principles of Bioastronautics, 133-138; and C. Frederick Hansen, "The Characteristics of the Upper Atmosphere Pertaining to Hypervelocity Flight," Jet Propulsion, XXVII (Nov. 1957), 1155-1156.X
- Emme, Aeronautics and Astronautics, 77, 82.X
- On Unidentified Flying Objects see several works by Donald E. Keyhoe, especially Flying Saucers in Outer Space (New York, 1953); and Donald H. Menzel, Flying Saucers (Cambridge, Mass., 1953).X
- Malcolm D. Ross, "A Consideration of the U.S. Navy Strato-Lab Balloon Program and Its Contributions to Manned Space Flight," in Proceedings of the Second National Conference on the Peaceful Uses of Space, Seattle, Washington, May 8-10, 1962, NASA SP-8 (Washington, 1962), 261.X
- Gerathewohl, Principles of Bioastronautics, 475-476.X
- David G. Simons, "The 1954 Aeromedical Field Laboratory Balloon Flights: Physiological and Radiobiological Aspects," Journal of Aviation Medicine, XXVII (Apr. 1956), 100-110; Simons interview; Bushnell, "Major Achievements in Space Biology at the Air Force Missile Development Center," 2-12.X
- David G. Simons, "Psychophysiological Aspects of Manhigh," Astronautics, IV (Feb. 1959), 32-33; Bushnell, "Major Achievements in Space Biology at the Air Force Missile Development Center," 27-34.X
- David G. Simons, "Manhigh II," Technical Report 59-28, Air Force Missile Development Center, Holloman Air Force Base, N. Mex., June 1, 1959; Simons, "Psychophysiological Aspects of Manhigh," 33, 62; Simons, "Observations in High-Altitude, Sealed-Cabin Balloon Flight," in Gantz, ed., Man in Space, 133-160; Simons et al., "Personal Experiences in Space Equivalent Flight," in Flaherty, ed., Psychophysiological Aspects of Space Flight, 39-41; Bushnell, "Major Achievements in Space Biology at the Air Force Missile Development Center," 34-41; Simons, "Space Medicine - the Human Body in Space," monograph No. 6, Journal of the Franklin Institute Series, Dec. 1958, 169-178; Simons interview. For a popularly written personal account of the Manhigh II flight see David G. Simons and Don Schanche, Man High (Garden City, N.Y., 1960).X
- "Manhigh III: USAF Manned Balloon Flight into the Stratosphere," Tech. Report 60-16, April 1961; Simons, "Psychophysiological Aspects of Manhigh," 63; Simons et al., "Personal Experiences in Space Equivalent Flight," 41-43.X
- Simons, "Observations in High-Altitude, Sealed-Cabin Balloon Flight," 146. See also Simons, "Manhigh Balloon Flights in Perspective," in Proceedings of the Second National Conference on the Peaceful Uses of Space, 243-248. Simons' record for a manned ascent stood until May 4, 1961, when Cdr. Malcolm Ross and Lt. Cdr. Victor G. Prather reached 113,740 feet in an Office of Naval Research Strato-Lab High V balloon, launched from the carrier Antietam. Prather was killed during helicopter recovery when he stood up in the "horse collar" sling and fell into the ocean.X
- Simons, "Manhigh II," 272-294; Hanrahan and Bushnell, Space Biology, 171-172; Simons interview.X
- Simons, "Observations in High-Altitude, Sealed-Cabin Balloon Flight," 137; Simons, interview. On Air Force solar flare observatories, see David Bushnell, "The Sacramento Peak Observatory, 1947-1962," Air Force Office of Aerospace Research, 1962.X
- Gerathewohl, Principles of Bioastronautics, 136-138; Hanrahan and Bushnell, Space Biology, 180-187; Connor, "Space Radiation Protection"; James A. Van Allen, "On the Radiation Hazards of Space Flight," in Benson and Strughold, eds., Physics and Medicine of the Atmosphere and Space, 2-11; House Committee on Science and Astronautics, 86 Cong., 1 sess. (1959), U.S. Aeronautics and Space Activities, Jan. 1 to Dec. 31, 1958: Message from the President of the United States, 3, 4.X
- Simons, "Space Medicine - the Human Body in Space," 162.X
- Connor, "Space Radiation Protection"; Hanrahan and Bushnell, Space Biology, 179-180, 187-188.X
- F. L. Van der Wal and W. D. Young, "Project MIA (Mouse-In-Able), Experiments on Physiological Response to Space Flight," Jet Propulsion, XXXI (Oct. 1959), 716-720; Ashton Graybiel, et al., "An Account of Experiments in Which Two Monkeys Were Recovered Unharmed after Ballistic Space Flight," Aerospace Medicine, XXX (Dec. 1959), 871-931; House Committee on Science and Astronautics, 86 Cong., 1 sess. (1959), Jupiter Missile Shot - Biomedical Experiments, Hearings; David S. Akens, Historical Origins of the George C. Marshall Space Flight Center (Huntsville, Ala., 1960), 52, 54-56; Gerathewohl, Principles of Bioastronautics, 98-108; Mae M. Link, Space Medicine in Project Mercury, NASA SP-4003 (Washington, 1965), 27-28.X