Chapter 4: The Maria
![Figure 57. - Location of photographs in this chapter; numbers correspond to figure numbers. [Base map courtesy of the National Geographic Society.]](./images/p66a.jpg)
![Figure 57. - Location of photographs in this chapter; numbers correspond to figure numbers. [Base map courtesy of the National Geographic Society.]](./images/p66b.jpg)
Areas of mare material occupy about 15 percent of the Moon's total surface. As shown in figure 14, most of them occur on the Earth-facing hemisphere. Mare areas are of two types, those that fill multiringed circular basins and those that fill irregular areas. The circular basins are believed to be impact features formed by the collision of giant meteoroids with the lunar surface; these were later filled to varying degrees by mare material. The basins lie at successively lower levels to the east, with Mare Smythii-the easternmost of the mare basins on the near side-lying almost 5 km below nominal lunar radius. The irregular maria lie in lowlands. The largest of these is Oceanus Procellarum, which lies on the west side of the Moon and is almost 2 km below nominal mean lunar radius.
Mare filling is characterized by several distinctive features that indicate a volcanic origin. These include many broad low domes with summit craters. Some of these domes closely resemble terrestrial basaltic shield volcanoes. In other areas, irregular and steep-sided volcanic piles dominate. Elsewhere, clusters of domes occur as in the Marius and Rumker Hills. Another type of feature is the broad lobate flow fronts that mark the edges of lava flows; these flow fronts extend several hundred kilometers in length and are as much as 100 m high. Other elongate flows closely resemble terrestrial flood basalts; samples returned by Apollos 11, 12, 15, and 17 confirmed this resemblance.
Other typical features on the maria are sinuous rilles and wrinkle ridges. Many sinuous rilles originate in craters near the higher margins of the mare basins and flow into the lowlands. Apollo 15 collected samples from the margins of Hadley Rille and confirmed the hypothesis that sinuous rilles are basaltic lava channels. Wrinkle ridges occur in all mare regions and form circumferential or medially transecting patterns.
Ages of the maria are being determined by two methods. Absolute ages are given by radiometric techniques. From these we know that the sampled lunar basalts are much older than their terrestrial counterparts. The basaltic lava flows range in age from 3.15 to 3.85 billion years, so the episode of lava filling on the Moon must have continued for at least 700 million years. Relative ages can be established by counting craters in mare surfaces. Comparison of crater counts on the lightly cratered lava flows in the northern part of Oceanus Procellarum with radiometric dates obtained for the basalts returned to Earth suggests that the Procellarum flows may be as young as 2 billion years. This date needs to be confirmed because it more than doubles the time of lava production.
Analyses of the returned samples show that the chemical composition of mare basalts varies across the Moon. These differences have also been correlated with the subtle color changes seen in spectral reflectance measurements; as a result, chemical variations can now be mapped far from the Apollo landing sites. - G.W.C. and H.M.
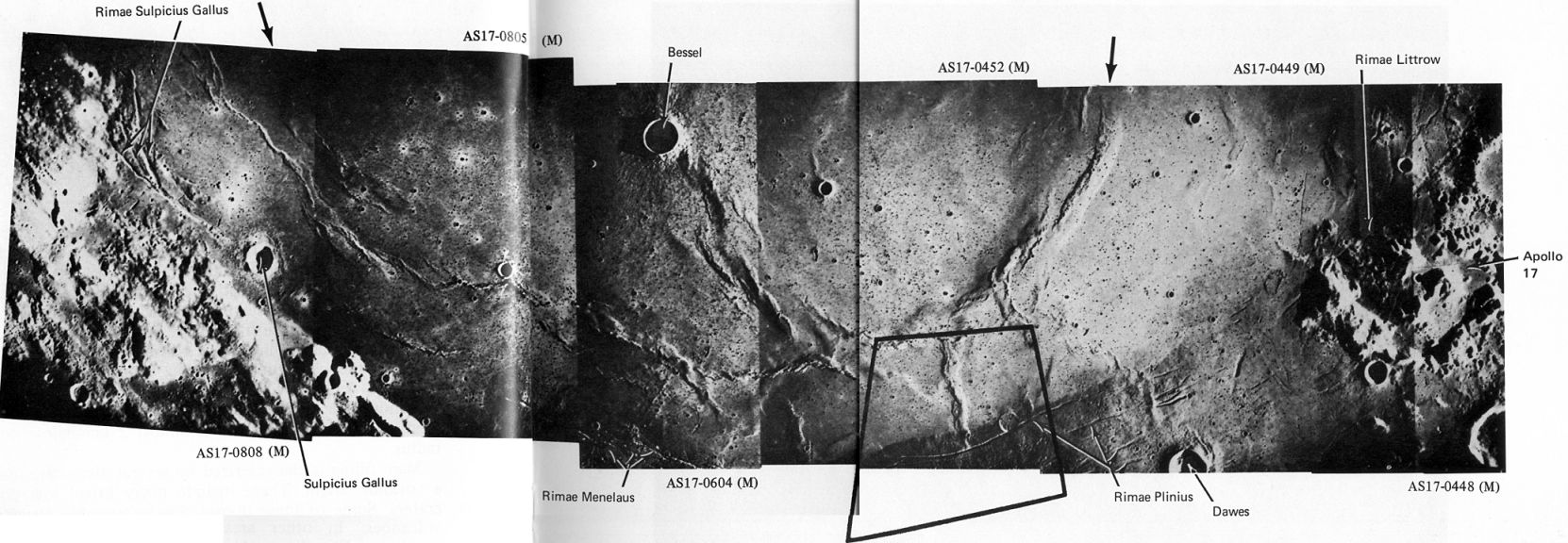
FIGURE 58. - This mosaic of Apollo 17 frames extends across southern Mare Serenitatis, one of the larger multiringed basins on the Moon's near side (fig. 14). The average diameter of the basin is about 680 km. Its generally circular outline is mimicked by the systems of arcuate rilles near the outer edge of the basin and also by the large system of mare ridges extending from arrow to arrow. Another feature of Mare Serenitatis is the nearly continuous ring of dark mare material that occupies the outer part of its floor. When these pictures were taken, the Sun angle was too low to show differences in albedo clearly. However, part of the ring of dark mare material is visible near the Plinius Rilles (Rimae Plinius) and the Littrow Rilles (Rimae Littrow). The stratigraphic relationships between the dark and light mare units are described in figure 59, which is an enlargement of the small area outlined in this figure. - G.W.C.
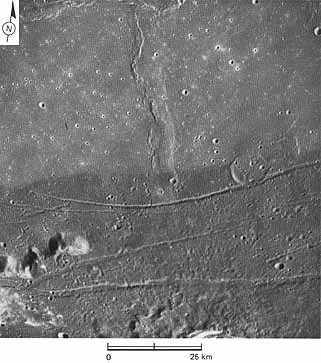
AS17-150-23069 (H)
FIGURE 59. - Some of the strongest tonal, color, and structural contrasts among mare materials occur in Mare Serenitatis. Accordingly, it has become a classic area for studying the sequence (or stratigraphy) of mare rocks. Earlier studies of telescopic photographs seemed to provide evidence that the lighter materials in the center of the basin (top half of this view) were emplaced before the darker lavas erupted along the basin margin. However, pictures returned by Apollo 17 show that the opposite is true. The dark materials were emplaced first. They were then tilted northward and broken by faults, such as those that bound the Plinius Rilles, before the light lava flooded against them (Howard et al., 1973). The large mare ridge or wrinkle ridge deforms both light and dark mare units but is much more prominent in the lighter unit. Detailed spectral studies and visual observations by the Apollo 17 astronauts show that the lighter-toned mare is relatively browner and the darker mare is relatively bluer. - K.A.H.
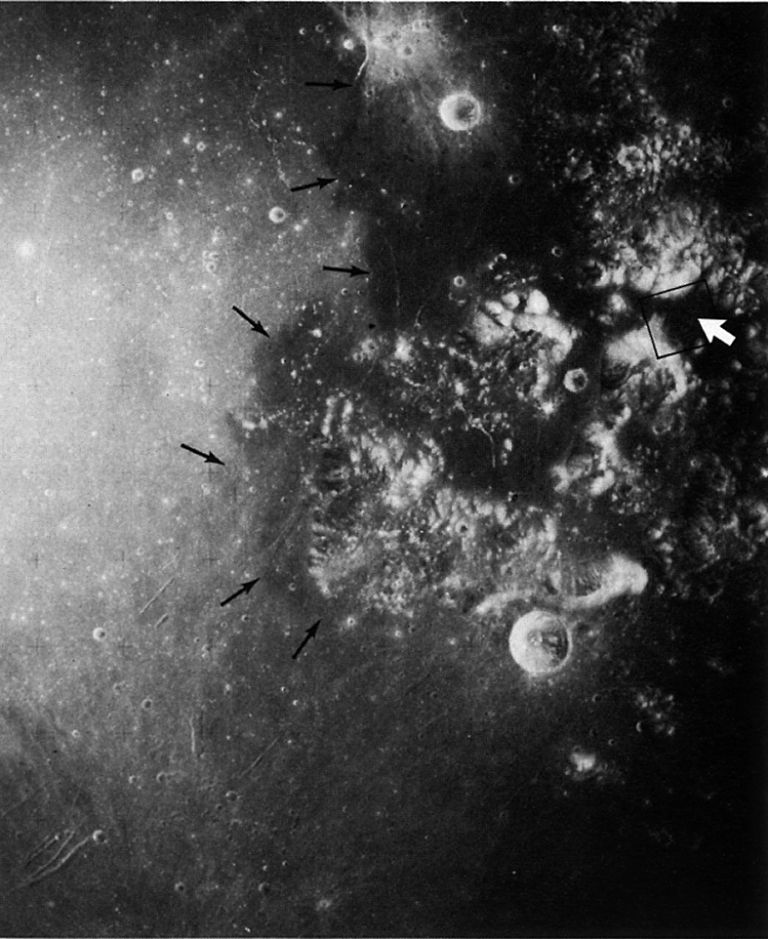
AS15-1115 (M)
FIGURE 60. - The southeastern margin of Mare Serenitatis and the surrounding Taurus-Littrow highlands are shown in this high Sun angle photograph. Also shown is the Apollo 17 landing site (large arrow) in a dark-floored valley between bright mountain massifs. The rectangle surrounding the landing site outlines the area covered by the two maps that follow in figures 61 and 62. The boundary between light mare material in the central part of the basin and the very dark mantling material surrounding the landing site area is indicated by several smaller arrows. The difference in albedo is much more pronounced in this picture than in the mosaic (fig. 58) at the beginning of this chapter because this picture was taken when the Sun was at a higher angle above the surface. Before the Apollo 17 landing, the dark material was interpreted to be a blanket of pyroclastic debris (volcanic cinders and ash). It was thought to be as young as Copernican in age (see fig. 13), and hence younger than most other mare materials elsewhere on the Moon. Analysis of samples returned from the Taurus-Littrow area has shown that while the dark material may be predominantly volcanic in origin, its age is considerably greater than had been predicted. The dark mantling material most likely consists of black and orange glass beads that form a layer on top of the valley floor basalt and are reworked into the regolith, thus causing the low albedo. - B.K.L.
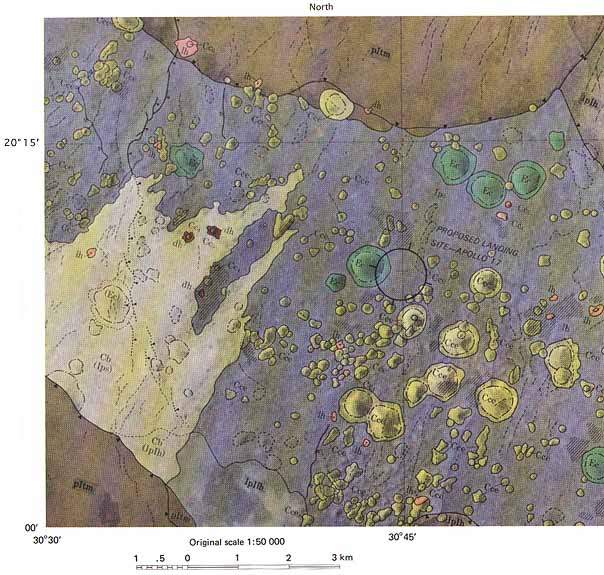
FIGURE 61. - This is part of a premission geologic map of the Taurus-Littrow area, compiled by B. K. Lucchitta (Scott, Lucchitta, and Carr, 1972) and published before Apollo 17 was launched. The actual landing point was very near the center of the large circle marking the proposed landing site. Letter symbols and colors designate the different types of rock materials and their relative ages as deduced from study of photographs available before the mission. Some refinements could now be made based on samples and data gathered by the astronauts on the surface and from orbital experiments. Apollo 15 panoramic camera photographs were the principal source of information for the original map, but mapping camera photographs, Orbiter pictures, and Earth-based telescopic pictures were also used.
On the explanation accompanying the map, each unit is identified and its relative position in the lunar time scale is shown. The explanation on the original map also included a description of the physical characteristics of each unit and a very brief interpretation of its origin and history. For example, unit pItm occurs on the steep hills north and southwest of the landing site and is interpreted to be composed of ancient rocks uplifted when the Serenitatis basin was formed. Unit Ips is a much younger, relatively smooth plains material that covers most of the Taurus-Littrow Valley. Before the mission it was interpreted as ejecta breccia or lava emplaced in a fluidized state; samples and other data gathered during the mission confirmed it was mare lava. Dark mantle material is shown by dot or line shading rather than by letter symbols and color. Throughout most of the valley it appears to be on top of (hence, younger than) unit Ips. It was interpreted as a blanket of pyroclastic debris. Unit Cb, bright mantle material, was interpreted as a deposit of avalanche debris derived from the steep mountain partly shown in the lower left corner of the map. - G.W.C.
|
|
. |
|
Dotted where buried; buried unit in parathensis. |
|||
|
|||
Bar and ball on downthrown side; dotted where buried
|
|||
|
|||
Line at base of slope, barb pointing downslope; solid where steep and high; open where gentle or low; may coincide with contact Interpretation: steep scarp in most places marks break in slope located near buried fault |
|||
|
|||
Groove, scarplet, ledge, or sharp break in slope Interpretation: slump scar, mass wasting trough, surface expression of fault, bedding plane, or trough between constructional ridges on Cb |
|||
|
|||
Craters > 500 m, old craters, crater remnants, and inferred craters |
|||
|
|||
Rimless or low rimmed Interpretation: degraded craters, graben remnants, and possibly volcanic craters; locally may be mass wasting, or drainage, pits along faults
|
|||
|
|||
Ih: light halo dh: very dark halo Small circle or dot locates crater or pit Interpretation: excavated material, possibly locally volcanic material |
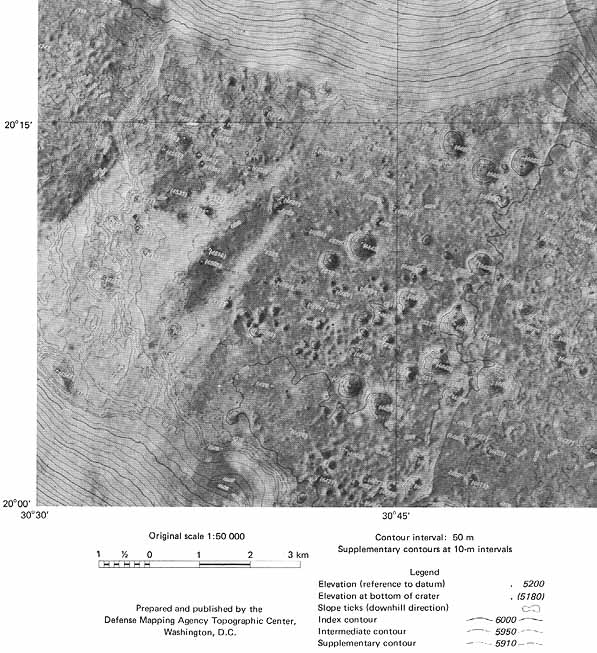
FIGURE 62. - This is a topographic contour map of the same area as the geologic map in figure 61. Topographic contour lines in red are superposed on an orthophoto base composed of rectified and mosaicked panoramic camera frames. The area shown is part of a larger map prepared by the Defense Mapping Agency Topographic Center and is included here to show the relationship between geology and topography. The steepness of the mountain slopes along the north edge and in the lower left corner is indicated by the closely spaced contours at 50-m intervals. These slopes are underlain by the very old rocks of unit pItm. The overall levelness of the valley floor-the area filled by younger rocks of unit Ips-is indicated by the widely spaced contours at 10-m intervals. An exception is the belt of closely spaced subparallel contour lines extending northward near the left edge of the map. These define an east-facing scarp or mare ridge interpreted on the geologic map as a fault. The average difference in elevation across the scarp is about 80 m, suggesting at least that much vertical displacement across the fault. The location and size of craters on the valley floor are shown by the many sets of circular contours. - G.W.C.
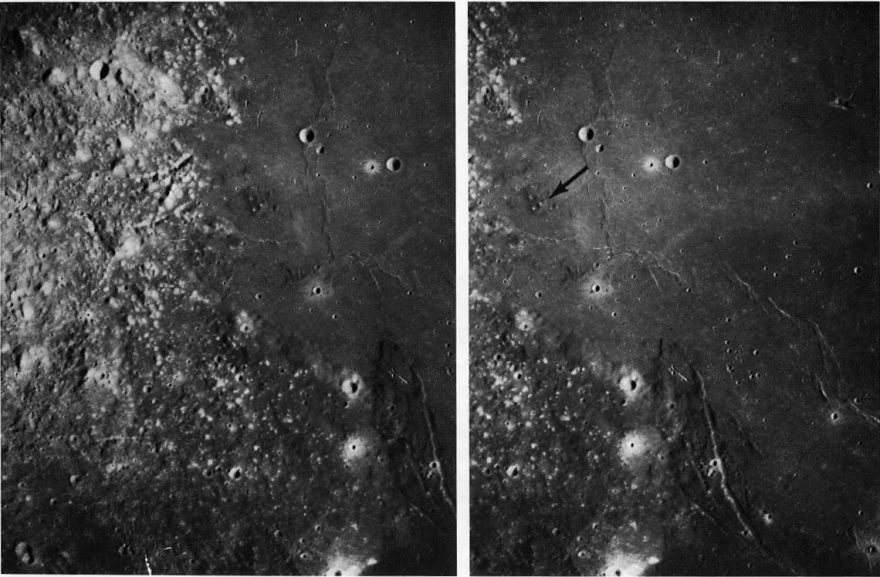
FIGURE 63. - This stereoscopic view shows southwestern Mare Serenitatis "lapping against" its shore of ancient highlands or terrae. The highlands near the Sulpicius Gallus rilles in the lower part of the picture are unusually dark-- darker even than the mare. M. H. Carr (1966) suggested from telescopic study that the darkness of the highlands is caused by a thin mantle of dark material, perhaps consisting of volcanic ash. The numerous small bright spots are knobs of highland material. They may have once been covered by the dark mantle but, if so, have since shed it. As elsewhere around the outer part of Mare Serenitatis, the rilles and the dark mantle in this area were originally thought to be younger than the lighter mare to the north. Apollo 17 photographs such as these have changed that concept. Now, the lighter mare is interpreted as embaying the faulted dark materials, just as in the Plinius rilles area (figs. 58 and 59). Isolated islands of dark mantled highlands that escaped inundation are shown by the arrow. - K.A.H.
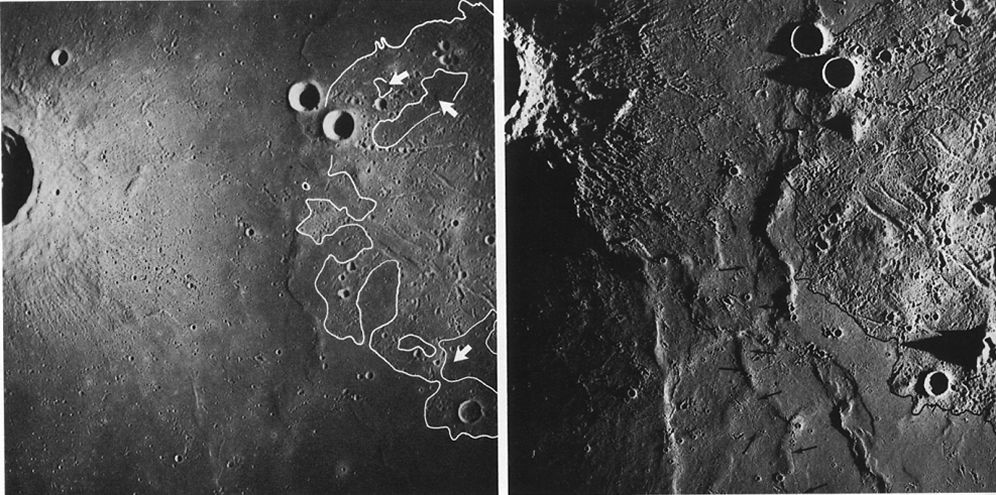
FIGURE 64. - These two contrasting pictures of the same area in southeastern Mare Imbrium were taken by Apollo 15, but on different revolutions under different lighting conditions. The picture on the left was taken when the Sun angle was 17°; the Sun angle was 2° when the picture on the right was taken. The large crater at the west edge is Timocharis. The area is dominated by three geologic units. The oldest is a fairly densely cratered fractured plains unit of moderate albedo that occupies the eastern part of the area. Next oldest is the mare unit in the central part, with its typically smooth, level surface and moderately low albedo. The youngest unit is the bright (high-albedo), highly textured ejecta surrounding Timocharis.
We have included the two pictures to illustrate the problems photogeologists sometimes face when drawing a contact line between units. The eastern edge of the mare is used as an example. Throughout most of the area shown the mare is in contact with the plains unit. Characteristically mare material is darker and smoother than plains material. Using the picture on the left in which albedo differences are enhanced because of the relatively high Sun angle, the contact might be drawn as shown. The line is equivocal in places, but, in general, it does satisfactorily separate darker areas from lighter areas. Using the picture on the right, in which surface relief is exaggerated because of very low Sun angle, the contact would be drawn as shown. Some areas dark enough to be mapped as mare in the first picture are here seen to be too roughly textured to be mare. As drawn, the line separates a unit that is both dark and smooth from a unit that is predominantly light and everywhere rugged.
Detailed stereoscopic examination of all available pictures of this area explains why some dark areas within the plains unit should not be classified as mare. In several of them there are structures resembling volcanic outlets (wide arrows on left photo). Similar structures were not found elsewhere within the plains unit. Therefore, it is likely that some if not all the darker areas of the plains are caused by veneers of dark volcanic ejecta so thin that the surface relief of the underlying plains is still visible.
An additional point of interest is the clearly defined sinuous rifle (small arrows in right photo) that extends half the length of the picture; the same rifle is almost invisible in the other picture. - G.W.C.
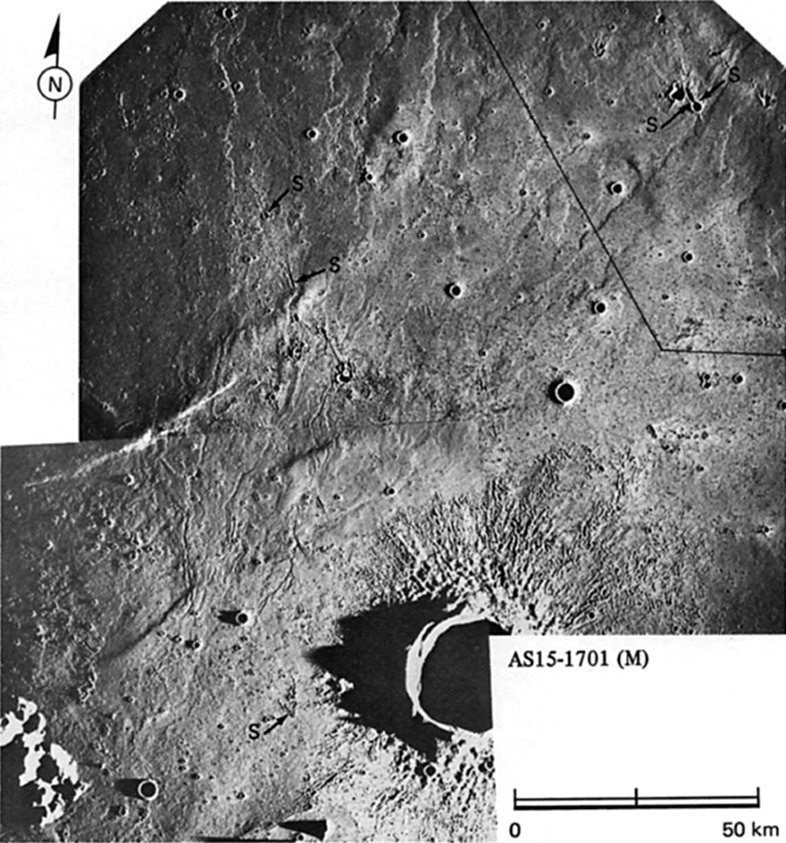
AS15-2295 (M)
FIGURE 65. - This photomosaic of an area of relatively young mare lavas in southwestern Mare Imbrium shows a complex of overlapping lava flows. The complex has been traced to its apparent source northeast of the mountain mass Mons Euler (formerly called "Euler B [Greek letter beta]") where the approximate location of a fissure has been deduced by detailed geologic mapping (Schaber, 1973). Individual flows are recognizable in this low-Sun (about 4°) picture as elongate lobes bounded by steep scarps. They are shown on the accompanying sketch map. Many contain one or more small rilles that are interpreted as flow channels. As individual flows are traced southward toward their source, they narrow and converge or terminate in the vicinity of the postulated fissure. South of the fissure distinct flow scarps are absent. A row of dark volcanic cinder cones along the southeast side of Mons Euler is alined with the postulated fissure, further strengthening the idea that this is an area of eruption. It is likely that the fissure is covered by its own lavas. The succession of geologic events in this area is easily decipherable. Secondary impact craters (as at S) from the large crater Copernicus overlie the lavas; hence the lavas are pre-Copernican, or Eratosthenian, in age. In turn the lavas have inundated part of the ejecta from the crater Euler; therefore, Euler is also pre-Copernican. Before Apollo pictures became available, it had been mapped as a Copernican crater. At the present time, only the lavas can be assigned an absolute age. About 2.5 billion years old (Schaber, 1973), they are older than all but a very few rock outcroppings in the entire United States. On the other hand, they are much younger than most, if not all, the samples collected on the Moon during the six lunar landings. Solid line shows position of lower left corner of figure 66. - G.G.S.
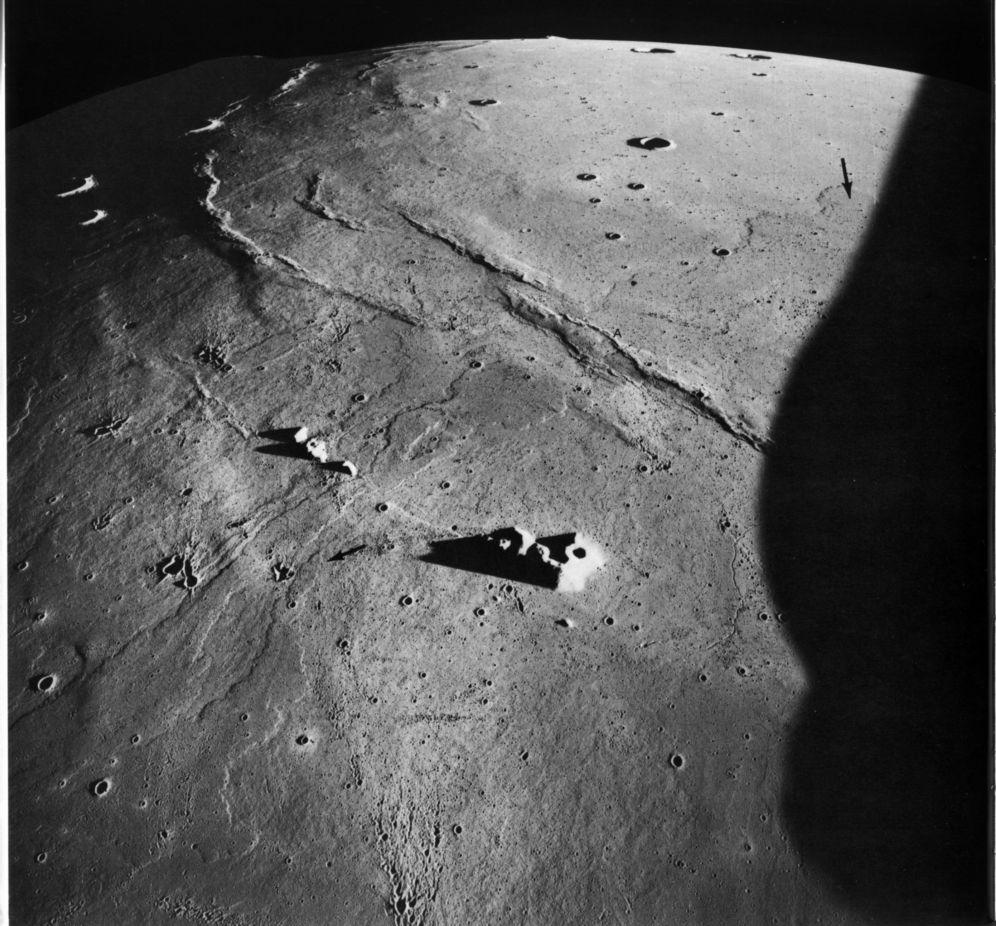
AS15-1556 (M)
FIGURE 66. - Two of the younger lava flows described in figure 65 are here followed northeastward to their terminations (see arrows) near the center of Mare Imbrium. The narrowness of the flows is somewhat accentuated by the obliquity of this view. From the postulated fissure vent near Euler B [Greek letter beta] to the farthest terminal lobe is about 420 km. The mare ridge at A must have been nonexistent or at least much more subdued at the time the flow passed that point, otherwise the flow would have ponded behind the ridge. The spray of irregular craters in the center foreground was formed by material ejected from Copernicus, 460 km to the south. - G.G.S.
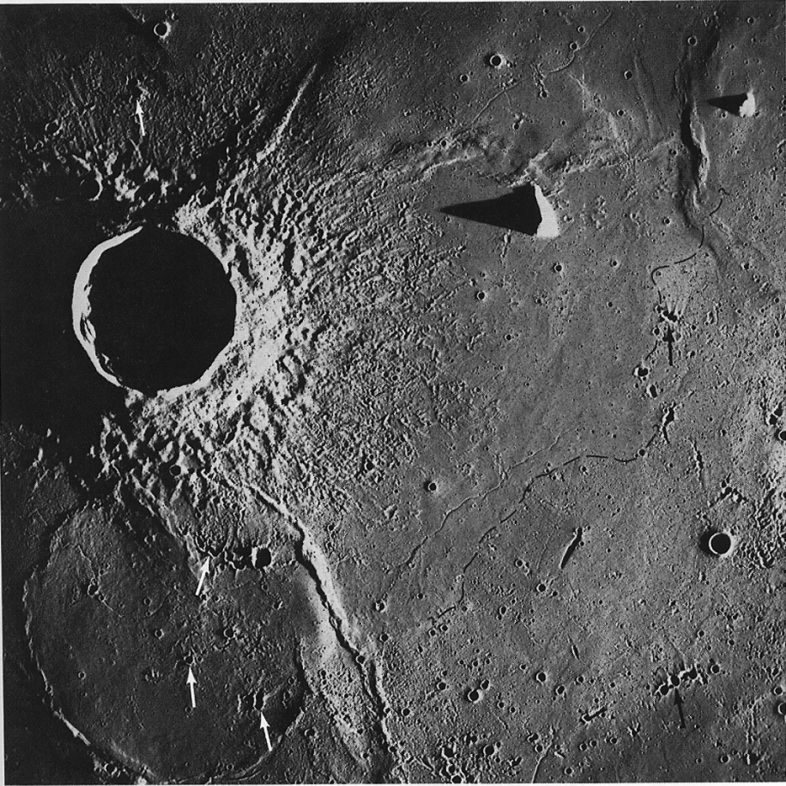
ASl5-1010 (M)
FIGURE 67. - This area is east of that covered in figures 65 and 66; the large crater is Lambert. Here also lava flows of more than one age are present. A sinuous band of smooth, sparsely cratered mare extends northeastward through the center of the picture. It is most certainly a young lava flow and contrasts strongly with the much more densely cratered older mare southeast of the dashed line marking the contact between the two. The western boundary of the young lava flow clearly laps upon and embays the blanket of ejecta deposits surrounding Lambert. Many radial ridges of ejecta and radial grooves or chains of secondary craters radiating outward from Lambert are faintly visible beneath the younger flow near its western boundary. These relationships prove that the younger flow postdates the formation of the crater. Many clusters of secondary craters from craters other than Lambert are present. The shape, orientation, and freshness of some (indicated by arrows) lead us to believe they were probably formed by ejecta from Copernicus, which lies 360 km further south. They are present on the older mare, on the ejecta from Lambert, and elsewhere around this area. However, none is present on the younger flow. If this observation is supported by further study, the younger flow must postdate even the crater Copernicus, and thus be younger than any other extensive lava flow recognized to date. - H.M.
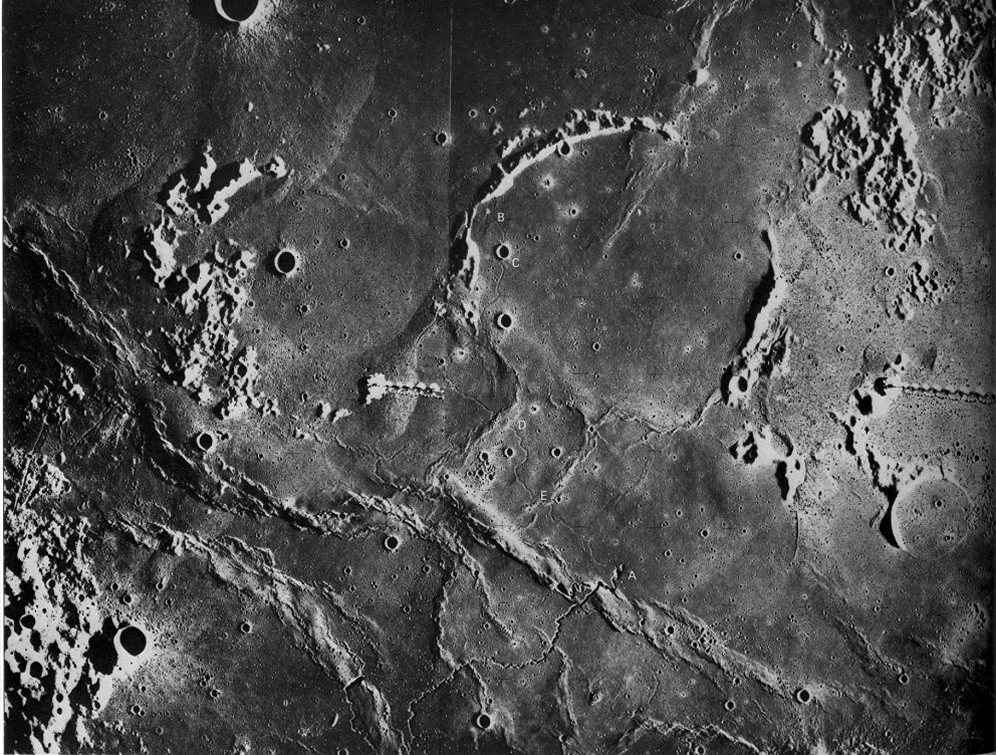
AS16-2839 (M) | AS16-2836 (M)
FIGURE 68. - Although this mosaic covers only a small part of Oceanus Procellarum, it shows a large number of different features that typify the mare surfaces of the Moon. All these features except the ubiquitous craters superposed on the mare are identified in the accompanying sketch map. The rugged terrae in the lower left corner mark the edge of Oceanus Procellarum. Similar terra materials project through the mare in many other places suggesting that the mare fill is thin here. Broad gentle arches, visible only in very low Sun pictures such as these, are numerous and seem to be independent of the even more numerous mare ridges or wrinkle ridges. The ridges are alined mostly northwest and to a lesser degree north-northeast. The alinement suggests-but does not prove-control by tectonic disturbance within the crust. A large forked rifle emanates from three elongate depressions located on or very near two of the largest ridges. This led Young (Young et al., 1972) to postulate that these rilles are lava channels or tunnels formed during "upwelling and outpouring of lava beneath a thin or viscous crust to form the mare ridges." The large rifle extends southward for 135 km beyond the picture. From the depression at A, a smaller rifle meanders about 100 km northward before disappearing at B. Note that this rifle is interrupted by a small crater (C) and by the two mare ridges at D and E. The crater is obviously younger than the rifle and, almost as certainly, so are the two ridges. Many small sinuous and arcuate rilles, small grabens, and faults are present. Although it is difficult to distinguish between some of them, most of these linear features are located on or near high areas of terra islands, mare arches, or mare ridges. Also identified on the map are the small possible volcanic domes. - G.W.C.
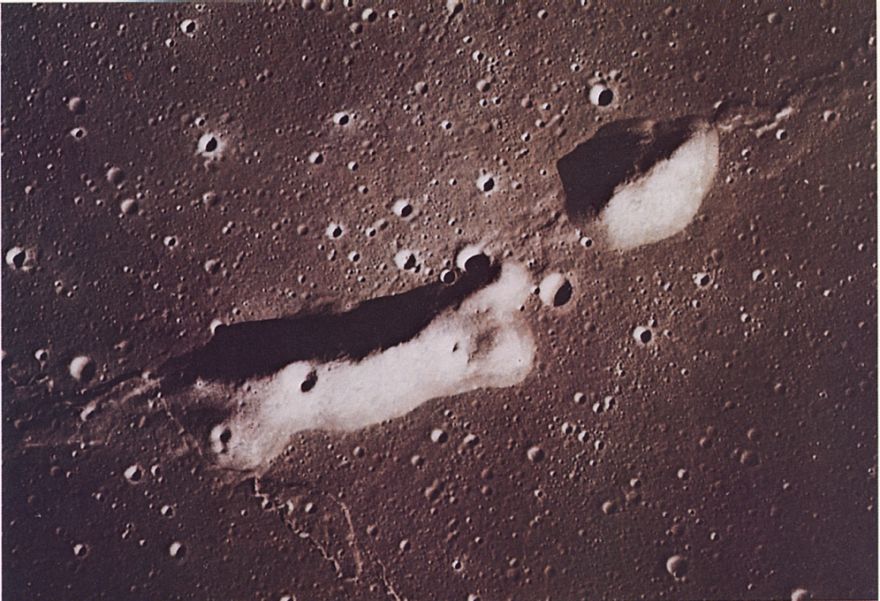
AS16-120-19244 (H)
FIGURE 69. - This cluster of features in Mare Cognitum was photographed in color with a handheld camera and 250-mm lens. Its location on a mare ridge is shown in figure 91. Thomas K. Mattingly, the astronaut who took the picture, noted the taluslike skirt of material around the base of this and many other positive relief areas in the western maria. In this photograph the apparent difference in tone (and in color on the original negative) between the hills and the slightly darker skirt of material around their base is largely, if not entirely, due to differences in slope-a more steeply sloping surface appears brighter, as in the walls of craters in the adjacent mare. However, a real color difference has been found at the base of other prominences. The suggestion has been made that in some mare basins the original level to which lava filled the basin has receded and that skirts such as these are, in effect, "bathtub rings" recording the higher level. The two hills pictured here are probably of volcanic origin. - M.C.M.
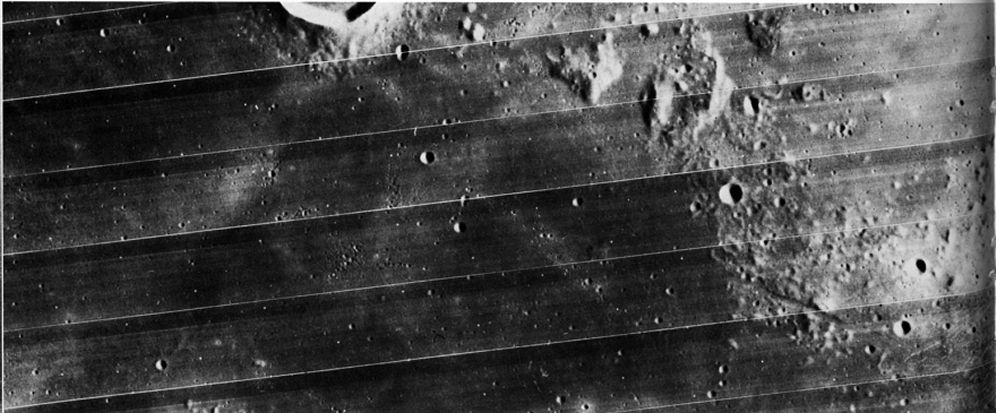
AS14-78-10378 (H) | AS14-78-10377 (H) | AS14-78-10376 (H) | AS14-78-10375 (H)
![FIGURE 70. - These two pictures were taken of the same mare area near the southeastern edge of Oceanus Procellarum, south and east of the crater Kunowsky. The lower left (facing page [bottom below]) picture is part of a Lunar Orbiter 4 high-resolution photograph taken when the Sun was at a moderate elevation of 18 to 20°.](./images/hrp80b.jpg)
L.O. IV-1334
FIGURE 70. - These two pictures were taken of the same mare area near the southeastern edge of Oceanus Procellarum, south and east of the crater Kunowsky. The lower left (facing page [bottom below]) picture is part of a Lunar Orbiter 4 high-resolution photograph taken when the Sun was at a moderate elevation of 18 to 20°. The picture below is a mosaic of Apollo 14 frames taken when the Sun was exceptionally low-0° to 2°. Douglas Lloyd designed the special experiment by which these near-terminator photographs were obtained, using very high-speed film in the Hasselblad camera.
The density of craters (more properly, the number of craters per unit area of surface) has been used by geologists as a tool to determine the relative age of rock units on the Moon's surface. The method has been applied principally in mare areas because crater populations generated on the irregular highland surfaces cannot be accurately measured. These two pictures illustrate some of the problems encountered when applying the method.
The number of craters that can be seen and hence counted is affected by the Sun angle. For example, many more craters are visible in the mosaic of low Sun Apollo pictures than in the Lunar Orbiter picture, and a detailed count of all craters in each picture would result in two radically different relative ages for the same area. Further, comparison of the two views shows that the apparent discrepancy in abundance of craters exists only among the very small craters-those a few hundred meters or less in diameter. The number of craters a kilometer or more in diameter is the same in both pictures. The explanation is that most small craters can be recognized as such only by the shadows they cast. The materials on their walls and rims are commonly indistinguishable from those of the surrounding terrain. For each picture there is, depending on the angle of Sun elevation, a threshold value of slope below which no shadow is cast. In the picture at lower left that value has been calculated to be about 5°. Consequently, those craters that have been degraded so that their slopes are less than 5° are not visible. In contrast, craters with slopes as gentle as 0.25° are visible in the picture below.
An observation immediately follows. Small craters have a relatively short lifespan. That is, once formed, they are rapidly degraded. Their rims are eroded, and their interiors are filled with debris from the continuing bombardment of the surface by other impacting bodies. By actual count about 80 percent of the small craters in this area have been so degraded that their slopes no longer exceed 5°.
It has also been shown (Soderblom and Lebofsky, 1972) that the small crater population here-and in most mare areas-is in a steady state. In other words, the rate of formation of new craters and the rate of destruction of existing craters (either by superposition of other craters, or by gradual erosion by much smaller craters) are balanced. It is fruitless, therefore, to count small craters because such counts will result in the same false age.
Fortunately, the crater counting method does yield satisfactory results when applied to larger craters. The same number of craters larger than about 1 km is visible in both pictures. This means that no craters this size or larger have been degraded to the extent that their slopes are less than 5°-as were 80 percent of the small craters. We may assume then that all the larger impact craters that ever formed on the upper part of the mare surface have been retained and that their relative abundances in different areas are a measure of the relative age of those areas.
With the careful application of this method, it has been possible to assign relative ages to most of the mare areas of the Moon. Using the absolute ages that have been determined for samples returned from the Apollo landing sites in the maria (Apollos 11, 12, 15, and 17), the relative time scale now has a quantitative base so that relative ages can be converted to absolute ages. - L.A.S.
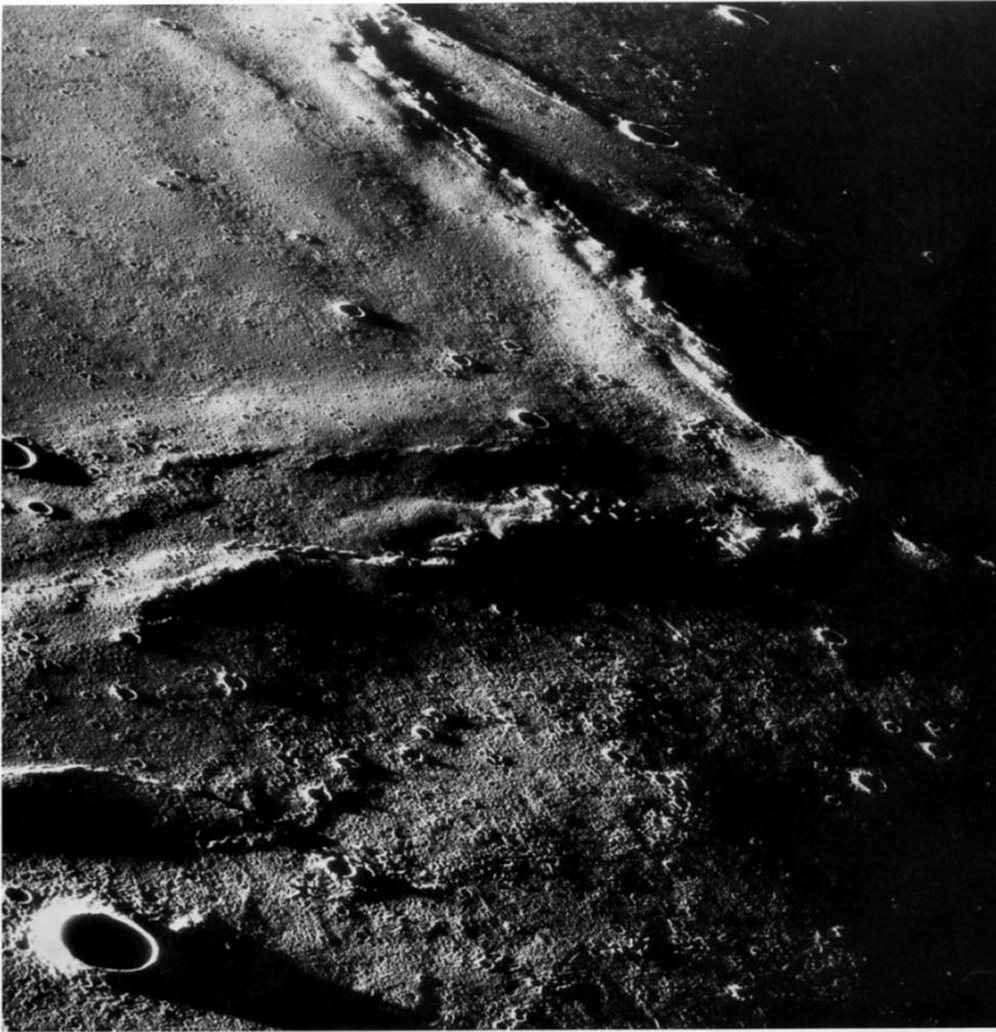
AS15-98-13361 (H)
FIGURE 71. - A very low Sun angle emphasizes detail in this south-looking oblique view of an area in western Oceanus Procellarum. The crater Seleucus is just outside the field of view to the right, and Seleucus E is in the lower left corner. The near-terminator view was exposed in a hand-held Hasselblad camera with a 250-mm lens. Low Sun illumination enhances small surface features and subtle differences in slope so that the broad, gently sloping arches associated with the mare ridges are clearly delineated. The oblique viewing angle accentuates the angularity that characterizes the path of many ridges as they are traced across the mare surface. (See, for example, fig.68.) - M.C.M.
![83] FIGURE 72. - Mare ridges in Oceanus Procellarum at the northwest tip of the Aristarchus plateau (lower right) are typical of mare ridges in many other areas.](./images/hrp83a.jpg)
AS15-2487 (M)
FIGURE 72. - Mare ridges in Oceanus Procellarum at the northwest tip of the Aristarchus plateau (lower right) are typical of mare ridges in many other areas. Among these are small crenulate wrinkle ridges (A) superposed on broad gentle arches (B), braided or en echelon patterns, a common tendency toward parallelism within limited areas, and a tendency to be deflected by obstacles such as the arcuate ridge belt of terra near the Aristarchus plateau. Measurements by photogrammetric methods have shown that mare ridges may project as much as 250 m (at C) above the surrounding mare surface. The origin of the ridges has yet to be explained satisfactorily by any single hypothesis. Among those proposed are (1) intrusion of deep-seated, postmare dikes and laccoliths, with some extrusion; (2) compressional buckling caused by sagging of comical mare surfaces; (3) buckling at the fronts of lava flows; (4) autointrusion of molten subcrustal mare lava forming laccoliths and squeeze-ups; (5) thrust faulting; and (6) drag folding along deep-seated transcurrent faults. The diversity in morphologies and patterns of ridges suggests that several hypotheses may be required to explain all of them. - C.A.H.
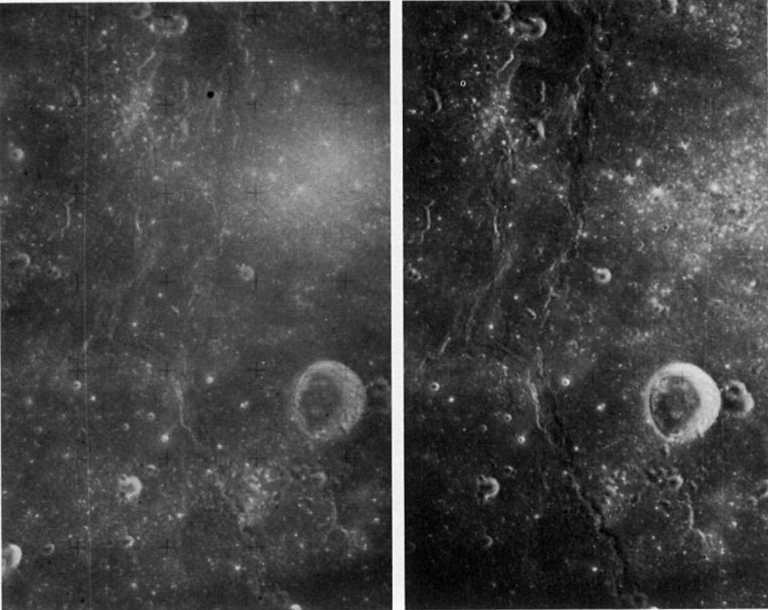
AS15-2401 (M) | AS15-2399 (M)
FIGURE 73. - Stereoscopic viewing, made possible by the overlap of Apollo mapping camera frames, does wonders for some lunar scenes. When viewed monoscopically, these photos taken at high Sun illumination in Mare Fecunditatis show bright patterns of craters and narrow mare ridges. Viewed stereoscopically, they reveal the relative depths of the craters; the sharp relief of the narrow ridges; an unsuspected broad, high swell between the parallel "ranges" of narrow ridges; and the even more surprising differences in elevation between mare surfaces in the left (west) and right parts of the photograph. The elevations of the mare surfaces probably mimic the variable depth of the buried floor of the Fecunditatis basin. The center of the basin, presumably the deepest part, is located near the left of the picture, where the mare surface is lowest. The large crater Messier G is 14 km in diameter. - D.E.W.
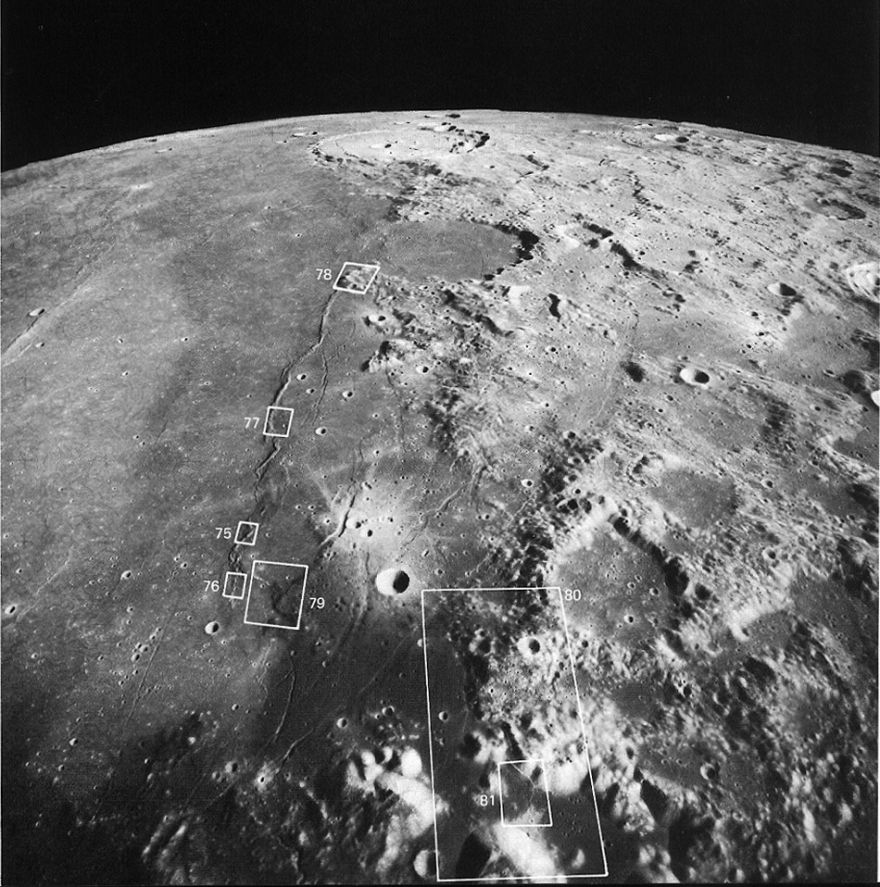
AS17-0939 (M)
FIGURE 74. - An oblique view looking northward along the east side of Mare Serenitatis. The Apollo 17 landing site (arrow) is near the lower edge. A nearly identical view was used earlier (fig.27), so the geology of the area will not be discussed again. We have included this picture here to serve as an index map for the next seven pictures, which are detailed views of parts of the Mare ridges visible in this photograph. -G.W.C.
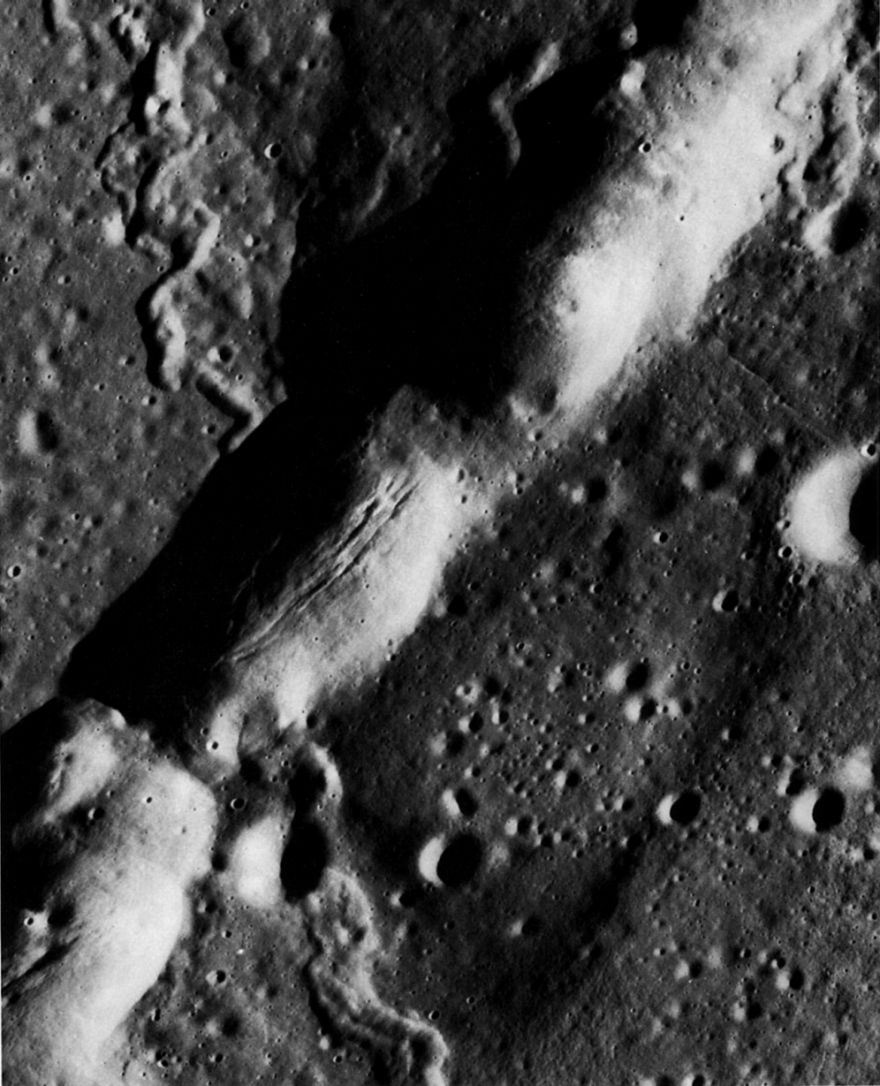
AS17-2313 (P)
FIGURE 75. - This and the next six pictures are enlargements of specific areas to show the detailed form of the mare ridges seen at much smaller scale in figure 74. Here can be seen a small segment of the most prominent ridge enlarged about 45 times. When seen in detail, the ridge is spectacularly crisp and well formed. The main ridge here is shaped like a string of sausages. Smaller wormy ridges appear on either side. Cracks in the top of the ridge probably formed by buckling of the mare lavas. As the following figure show, most other parts of the ridge are less symmetrical than this part. - K.A.H.
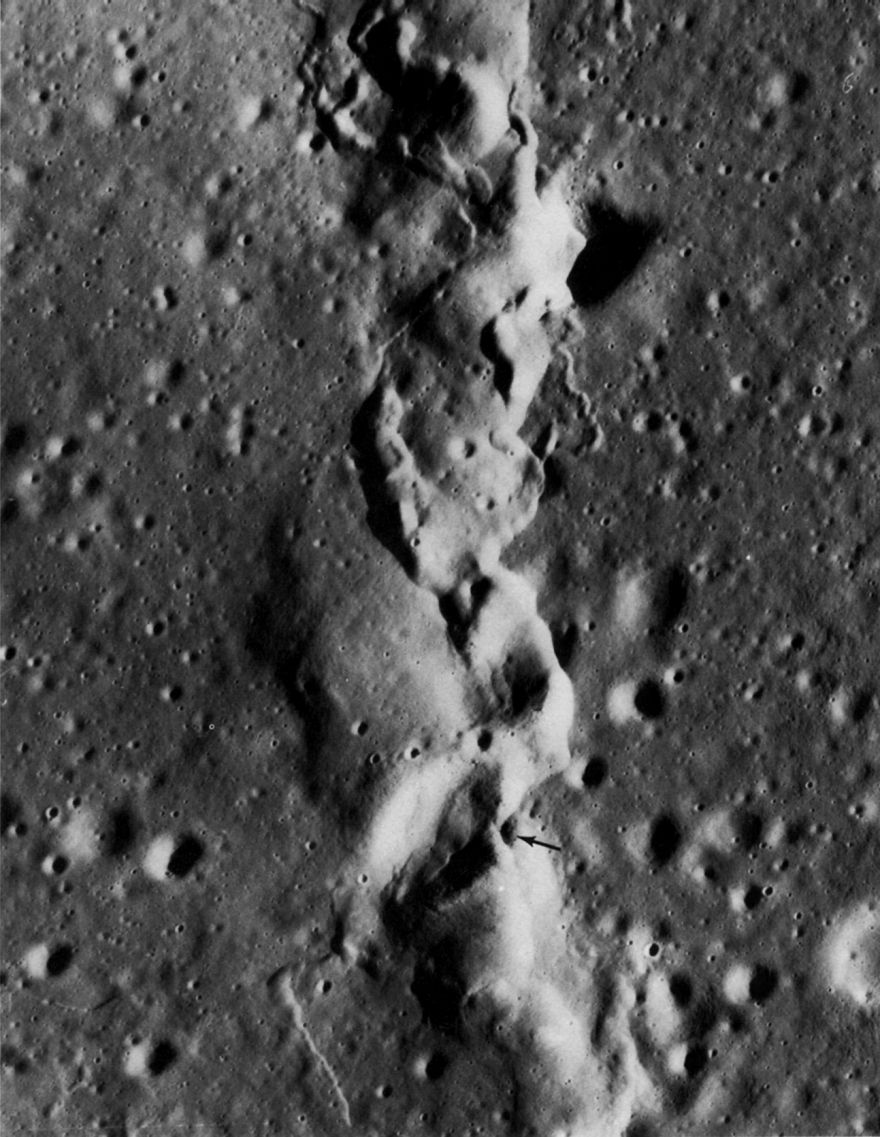
AS17-2313 (P)
FIGURE 76. - This part of the ridge appears to have flowed over the ground to the right. A careful search will reveal several craters overridden by the ridge along its right edge. A large oblong crater on the ridge near the bottom of the photograph has evidently been distorted by the ridge; in fact, the right edge of the crater seems to be cut off. (See arrow.) One can imagine that a sheet of soft putty might form a similar ridge if it were thrust over to the right. Model experiments and observations of deformed rocks on Earth have shown that large masses of rocks, even hard rocks like basalt, can behave like putty over sufficiently long intervals of time. - K.A.H.
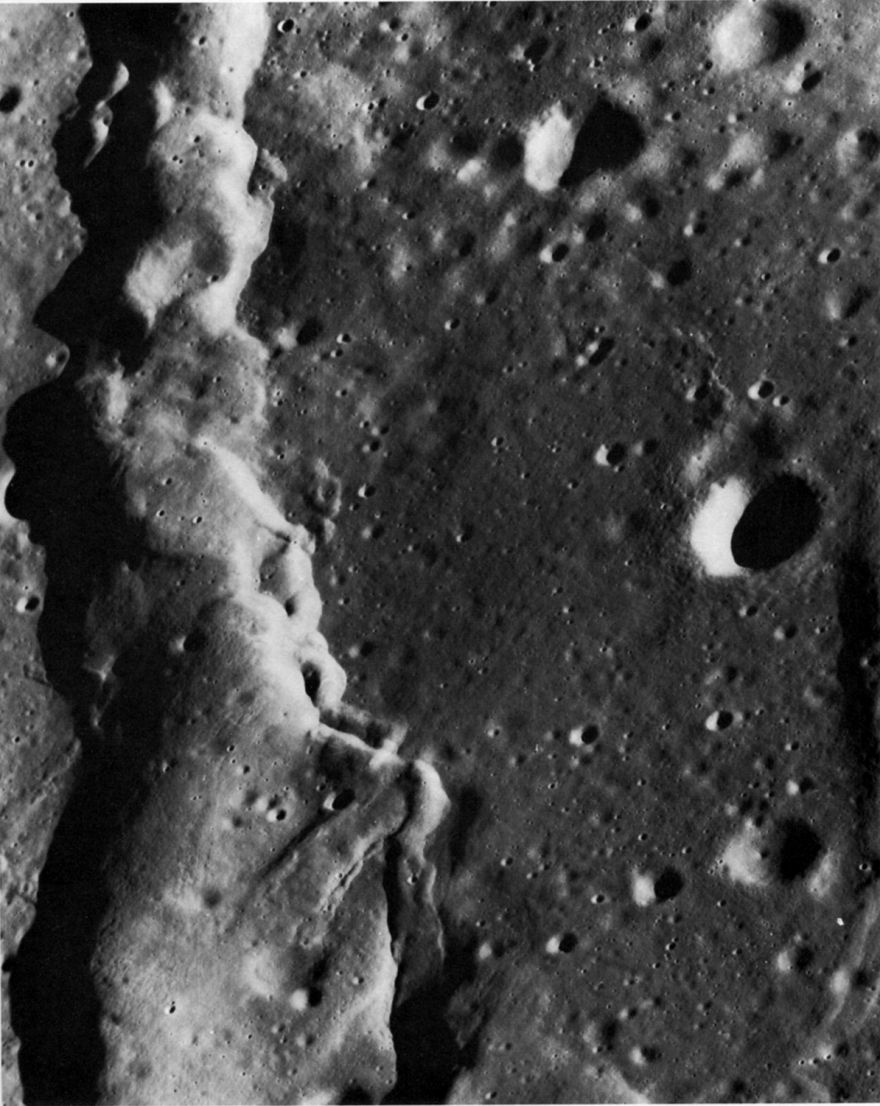
AS17-2313 (P)
FIGURE 77. - Here again the ridge has overridden craters along its right side. Many other mare ridges that are older may once have looked like this. The freshest examples of lunar features, like this one, are the best places to look for hints on origin. - K.A.H.
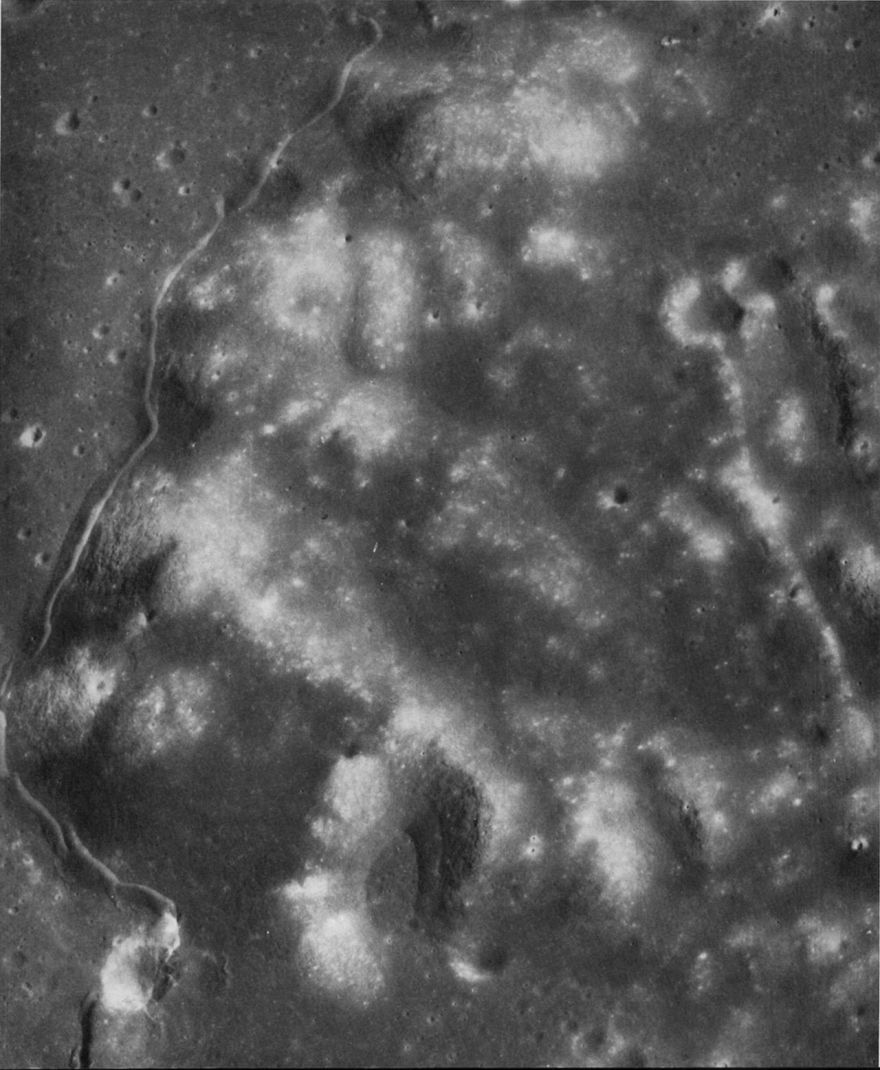
AS15-9298 (P)
FIGURE 78. - At its north end of the ridge becomes a scarp that wraps around the base of the highlands like a shoved rug. Were the mare lavas thrust against the highlands? This might seem easiest to imagine if the lavas had been only partly solidified when they were deformed, but the lavas had had plenty of time to solidify. The ridge deforms numerous impact craters, such as the one near the bottom of the photograph. A long time-probably hundreds of millions of years- had to pass for these impact craters to form after the lavas crusted over and before the ridge formed. - K.A.H.
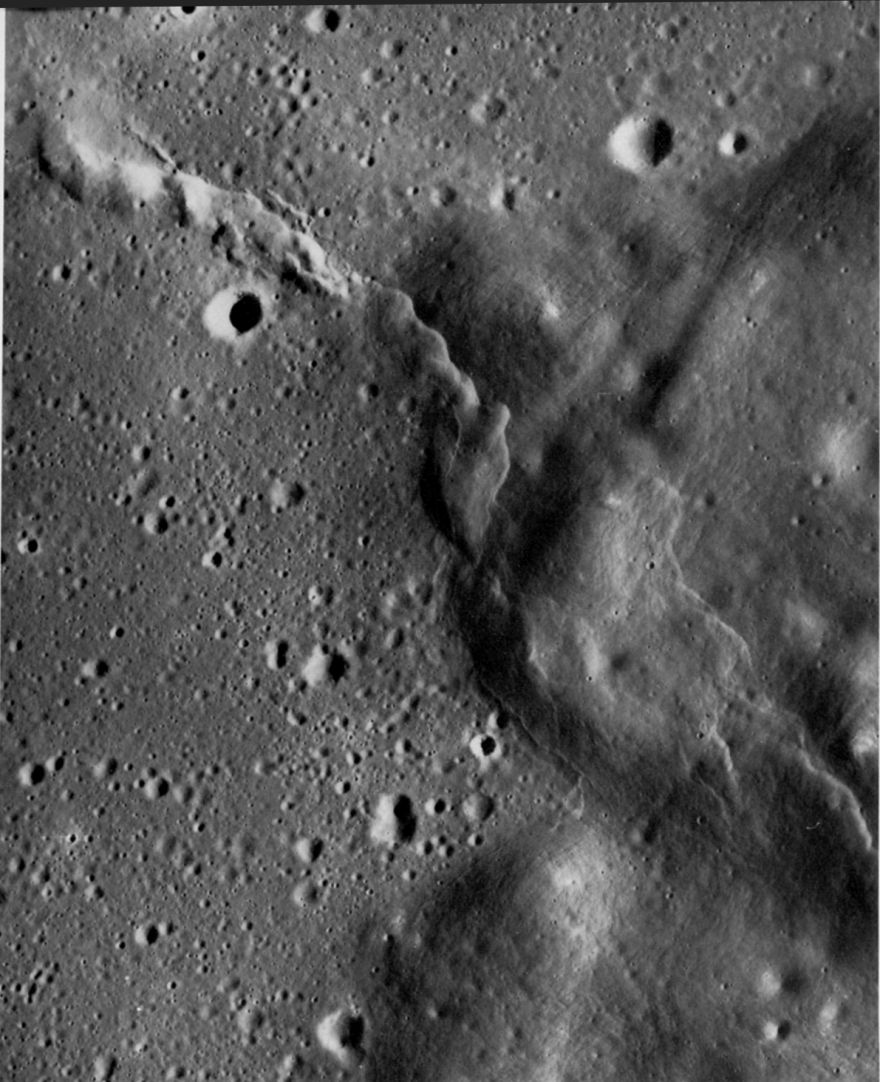
FIGURE 79. - In the upper left corner of the photograph, a lunar ridge heads in a southeasterly direction toward the dark mantling unit that rings Mare Serenitatis. At its south end, one branch of the same ridge disappears in the dark mantling unit. The ridge boundaries are crisp and clear in the lighter mare materials in the left half of the photograph. However, in the much darker and topographically higher unit to the right, the ridge is subdued. (Owing to especially favorable lighting conditions, the true height of the east-facing scarp of the ridge is exaggerated.) The sequence of events worked out from the study of this area is as follows: (1) the Serenitatis basin was probably formed by the impact of a giant meteoroid; (2) the rim materials, and perhaps also the inner part of the basin, were flooded by dark basaltic lavas and associated volcanic (pyroclastic) debris; (3) the central part of the basin was filled by a lighter colored basaltic unit; and (4) gravitational adjustments to the enormous mass of volcanic fill probably caused the formation of the ridges in the light mare in the inner part of the basin and locally in the dark outer ring. - F.E.- B.
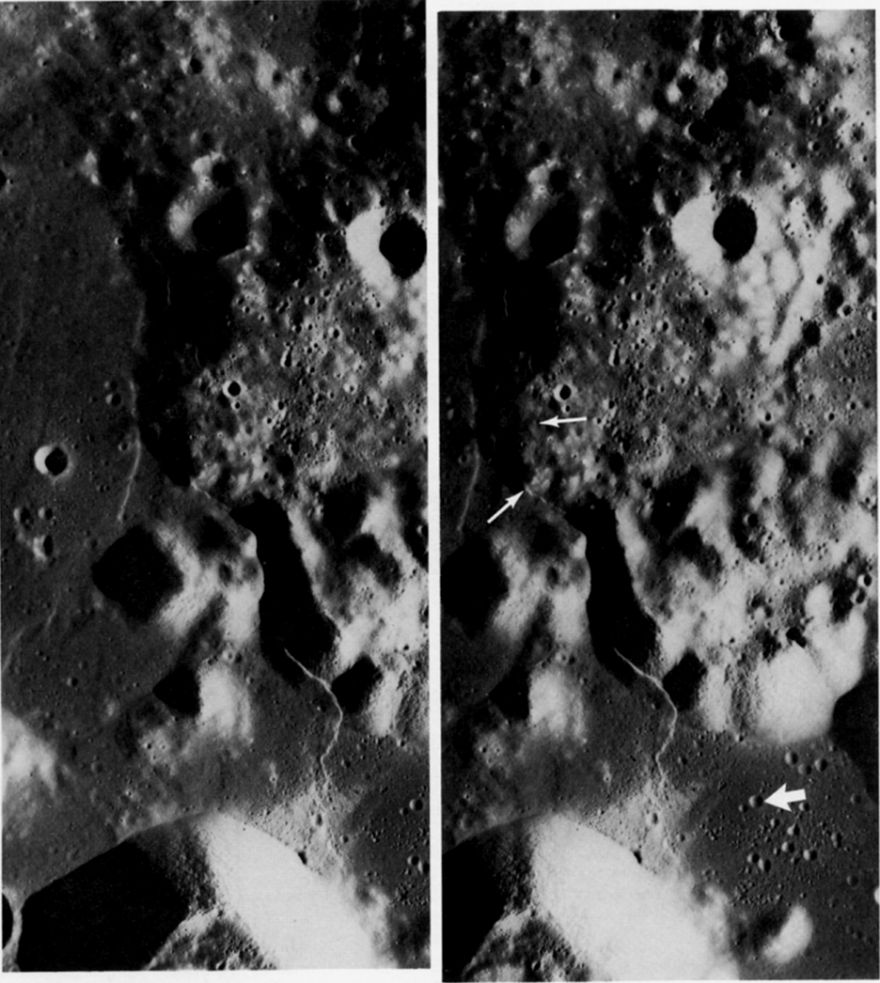
AS17-2314 (P) | AS17-2309 (P)
FIGURE 80. - A stereoscopic view of the only mare ridge climbed by man. The ridge crosses the Taurus-Littrow valley shortly west of the Apollo 17 landing site (large arrow) and was traversed by astronauts Gene Cernan and Jack Schmitt in the Apollo 17 lunar roving vehicle. Geologic and topographic maps of the area appeared earlier in this chapter (figs. 61 and 62). From the valley floor the ridge extends northward into the highlands. It is offset between the two small arrows and is partly buried by dark mantling material along its northern extension. - B.K.L.
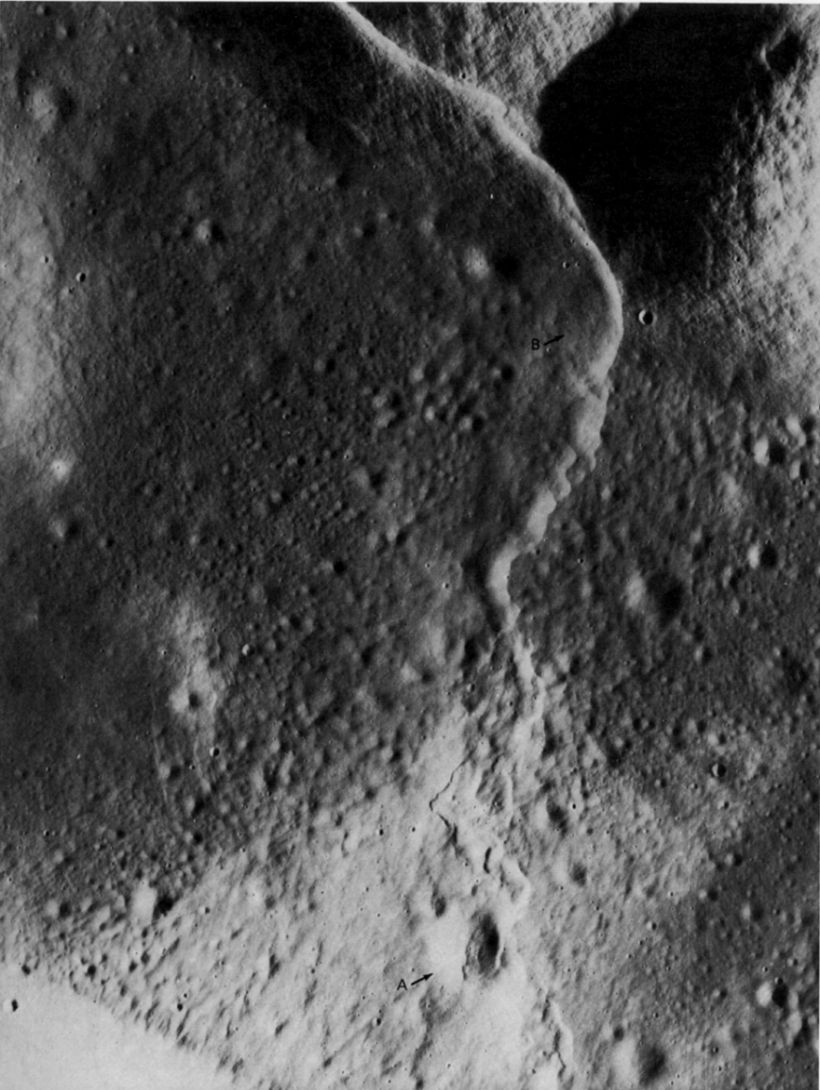
AS17-2309 (P)
FIGURE 81. - This enlargement provides a closeup view of part of the ridge. The fact that a fairly young impact crater (A) is deformed by the ridge and the generally fresh appearance of the ridge both suggest that it is fairly young. Within the valley, the ridge consists primarily of east-facing imbricate scarps, although west-facing lobate scarplets are also present. After crossing into the highlands (B), the ridge becomes a simple one-sided scarp resembling a fault scarp. If faulting was involved, movement was upward on the left side or, less probably, downward on the right. The Apollo 17 astronauts were unable to provide conclusive answers concerning the origin of the ridge owing to the thick cover of regolith and avalanche material. Photogeologic study indicates that part of the ridge may have formed by displacement along a fault or, less likely, by the upwelling of lava. - B.K.L.
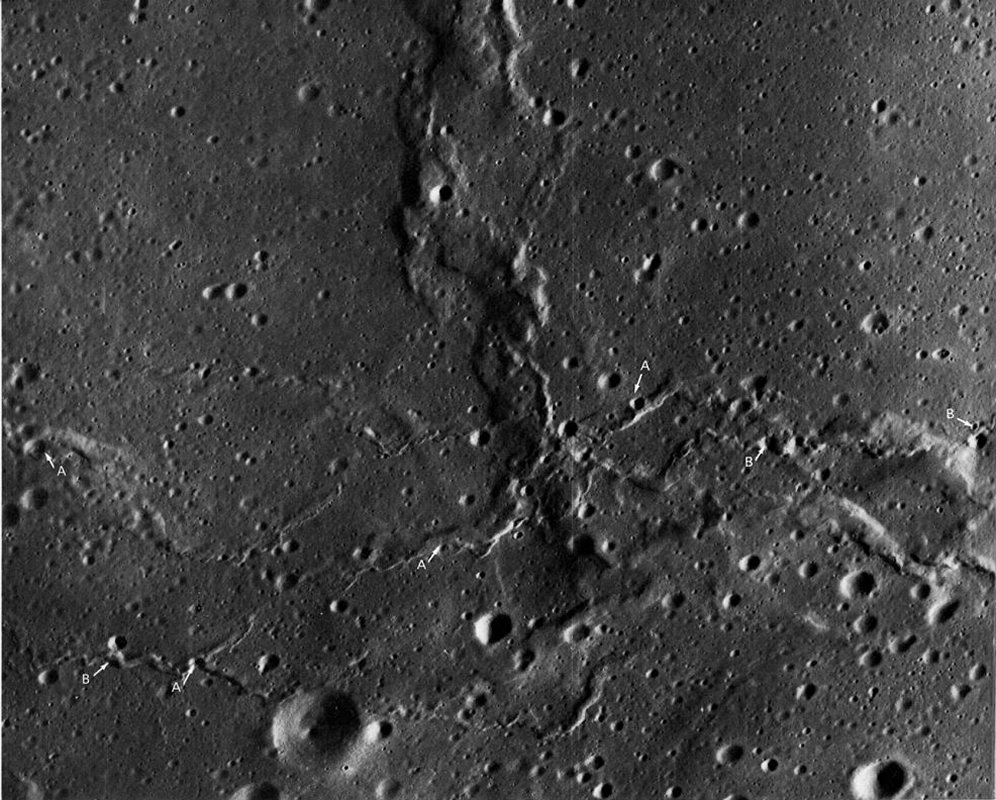
AS15-9361 (P)
FIGURE 82. - These intersecting sets of mare ridges are near the western edge of Mare Serenitatis. One set of generally small, sharply defined lobate scarps and ridges trends irregularly east to west. It cuts across a set of broader, more subdued ridges that trends north to south. On the basis of morphology and crosscutting relationships, the first set appears to be the younger of the two. Study of the better preserved younger set provides two clues to its origin. In several places (designated by A) incomplete craters are present on the flanks of ridges, but the other parts of the originally circular craters are absent on the adjacent mare floor. This suggests that some segments of the ridges are the frontal edges of thrust plates that have ridden over an already solidified and cratered surface. In several other places (B) craters on the mare floor appear to be partly overlapped by lobed projections of the ridges, suggesting inundation of the craters by a viscous material. The ridges in this area as in others may thus be the response of solidified rocks to faulting and possibly also to the movement of molten rocks. - B.K.L.
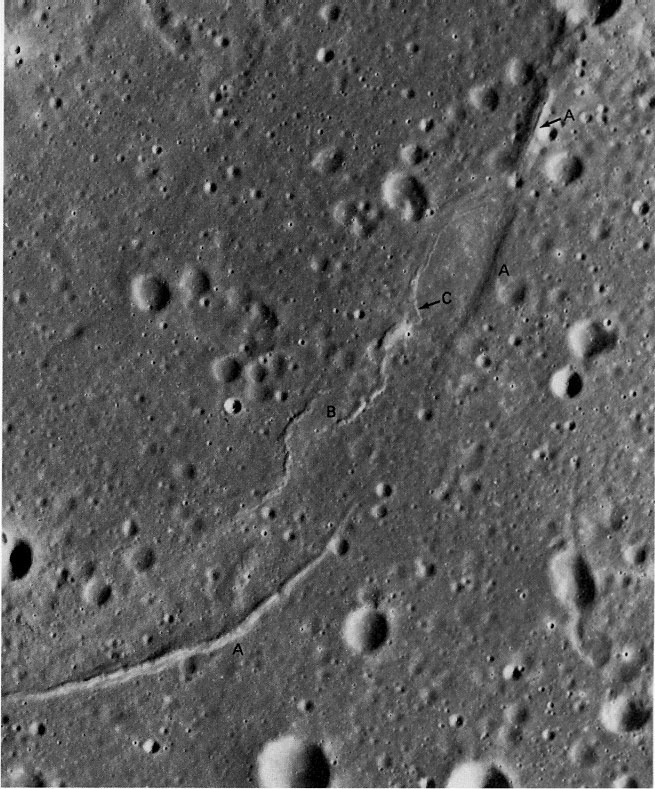
AS17-3075 (P)
FIGURE 83. - The arcuate ridges shown here are part of the large crater Lambert R, which is mainly deeply buried and now outlined by mare ridges and a few isolated remnants of the original rim. This is one of many examples in which underlying topography is reflected by mare ridges, thereby suggesting a causal relationship. The ridge segments at A are probably caused by solidification and sagging of the mare lavas over the crater rim. Crustal buckling caused by sagging of the lava inside the crater rim may have formed the inner ridges B and C. The exact origin of mare ridges is still debatable. - C.A.H.
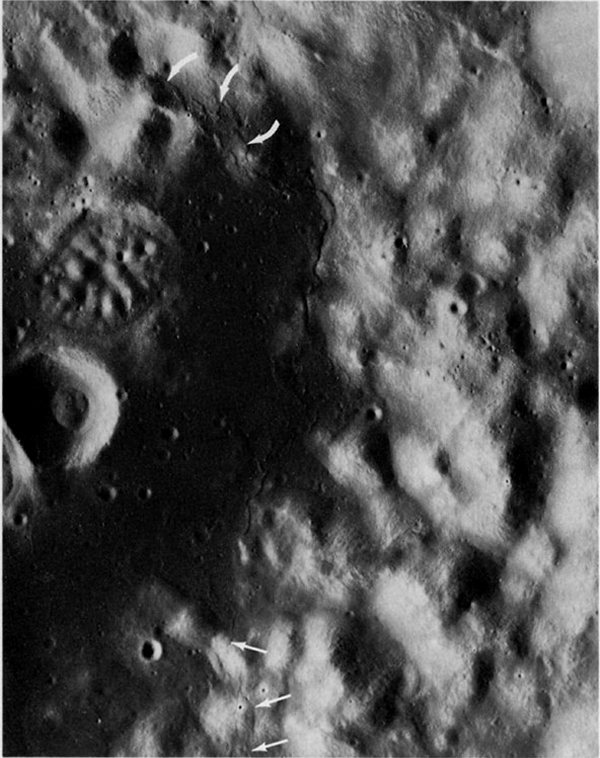
AS17-1913 (P)
FIGURE 84. - This picture is included for several reasons. It is an example of a mare ridge on the far side of the Moon. It also shows that mare ridges occur in small bodies of mare. Finally, it serves as another example of a mare ridge transecting terra as well as mare. The scene covers part of the floor and eastern wall of Aitken, a large (135 km) crater near the center of the far side. The dark area is mare material, forming part of Aitken's floor. The ridge along the east edge of the mare fill extends southward (straight arrows) for a considerable distance beyond the edge of the picture, gradually ascending the east wall of Aitken. To the northwest (curved arrows) the same ridge extends for about 7 km diagonally along the north wall before disappearing. Whether in the mare or in the terra materials of the crater wall, the morphology and geometry of the ridge suggest that viscous material was squeezed out along a fault (?) plane that dips gently westward. The oval area patterned like a turtle shell is probably volcanic in origin. Other examples of this rather rare feature are shown in the chapter entitled "Unusual Features." - G.W.C.
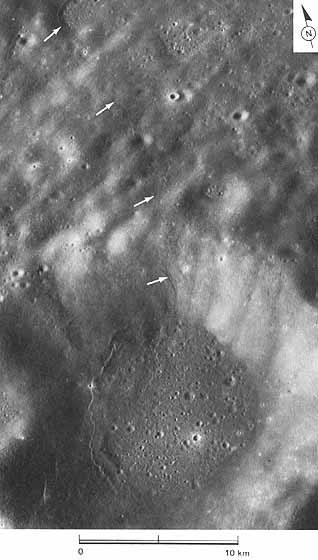
AS17-1676 (P)
FIGURE 85. - In this unnamed crater on the far side we see ridges closely resembling those already described. Inasmuch as no mare materials are present here, the term "mare ridge" is not appropriate. The materials occupying most of the crater floor, while resembling mare, are plains deposits. Those along the western part of the floor and the lower part of the west wall probably consist of unconsolidated erosional debris from the wall. The crisp appearance of the ridges as they transect unconsolidated material proves that they are relatively young and that they are composed of resistant material. Here also the ridges may be related to faulting. The ridge nearest the center of the crater can be traced (arrows) part of the way up the north wall; interrupted segments are visible along the same trend northward to the edge of the picture. They may mark a steeply dipping fault plane. - G.W.C.
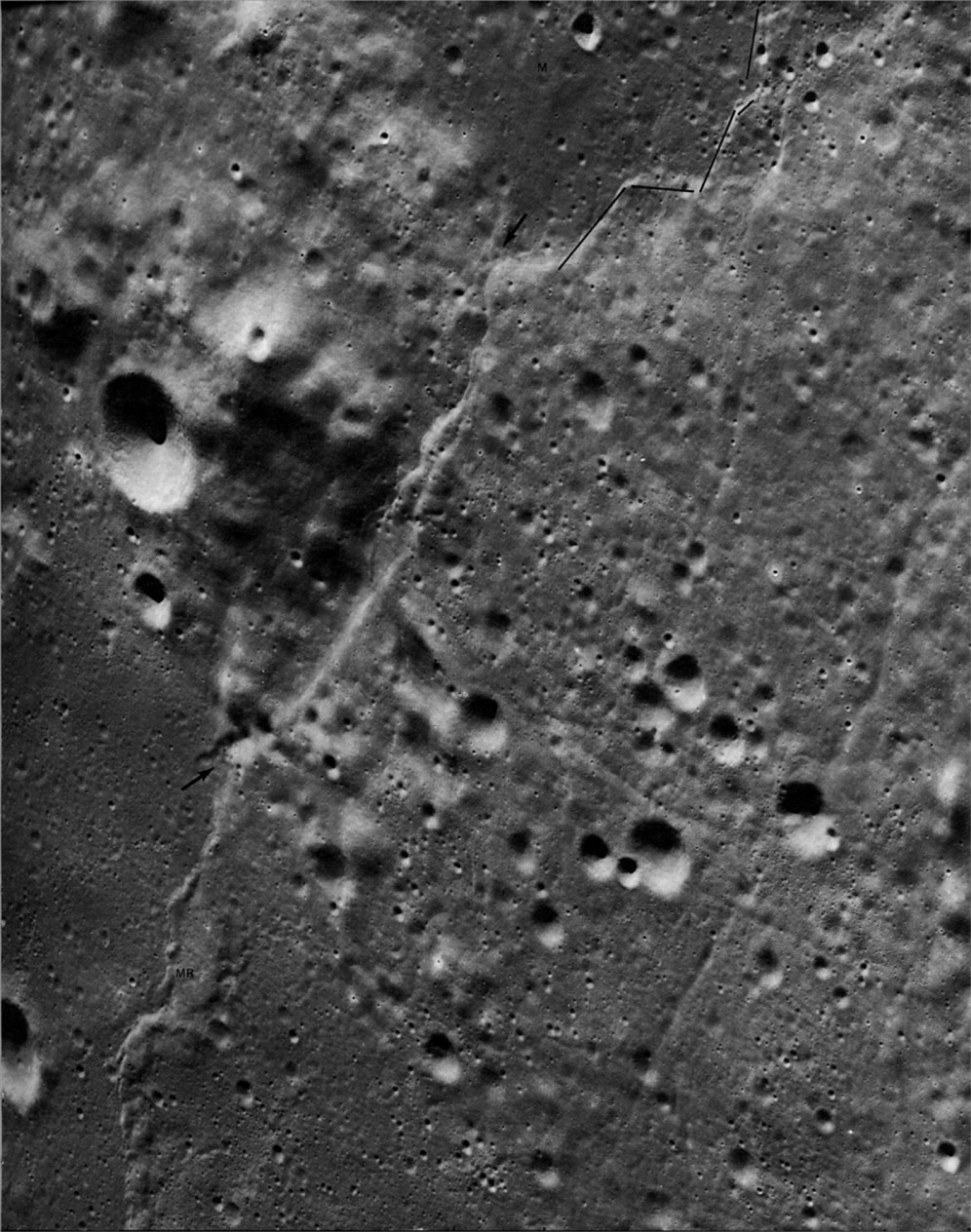
AS16-5452 (P)
FIGURE 86. - In this picture we see another example of the close relationship between mare ridges and faults. The area is in the Montes Riphaeus between Mare Cognitum and Oceanus Procellarum. A small part of Oceanus Procellarum is shown in the upper left. A mare ridge (MR) extends across Procellarum to the edge of the Montes Riphaeus. In all visible respects it is typical of a great many other mare ridges. Across the Montes Riphaeus (arrow to arrow) the trend of the ridge becomes a fault or series of faults causing the valley. Narrower valleys or grooves in the lower part of the picture are parallel to the larger valley and they too probably originated by faulting. Near the east edge of the picture the Montes Riphaeus are bounded by mare (M). Parts of the contact are quite angular (heavy lines), suggesting a series of offset faults, possibly continuations of the main mare ridge-fault trend. - G.W.C.
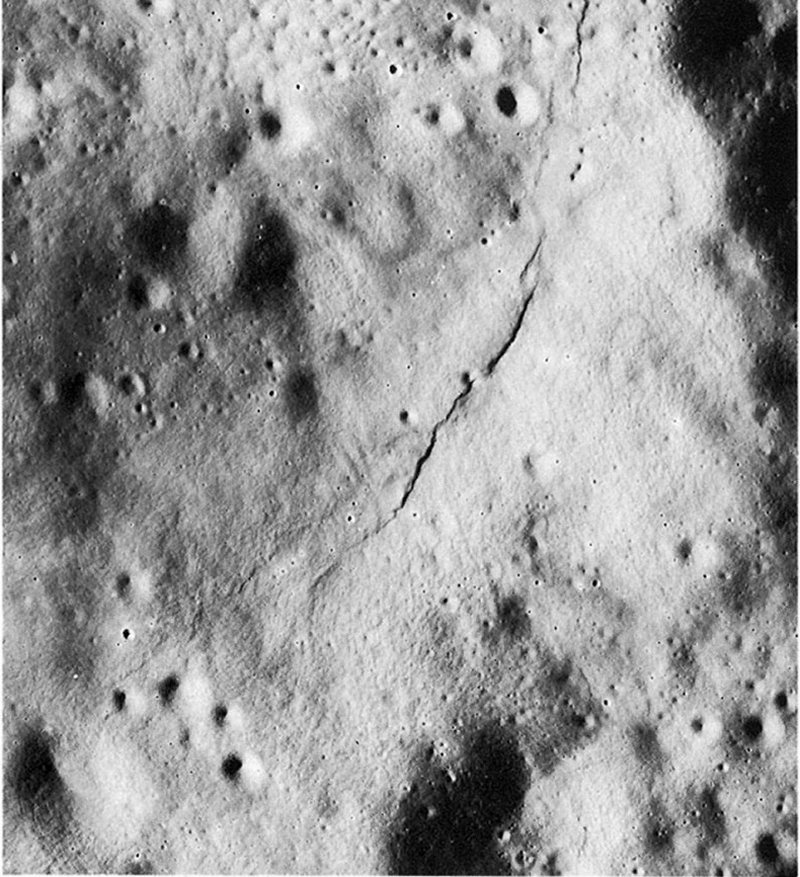
AS16-4970 (P)
FIGURE 87. - This crenulate lobate scarp occurs within a small depression on the far side. It is not related to any obvious crater source, although it is similar to lobate flow fronts within the ejecta blankets of some young impact craters. Although it resembles some mare ridges, it is clearly confined to highlands terrane. Small scarps of this kind- unrelated to any apparent source of material or to any detectable tectonic control-are widely scattered in the highlands; their origin remains enigmatic. Mass wasting along a fault scarp might have produced the lobate configuration. A flow of locally derived surficial debris is also a plausible explanation; in this case, such a flow might have been triggered by small impacts on a ridge to the northwest. - C.A.H.
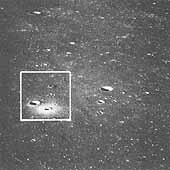
AS16-120-19237 (H)
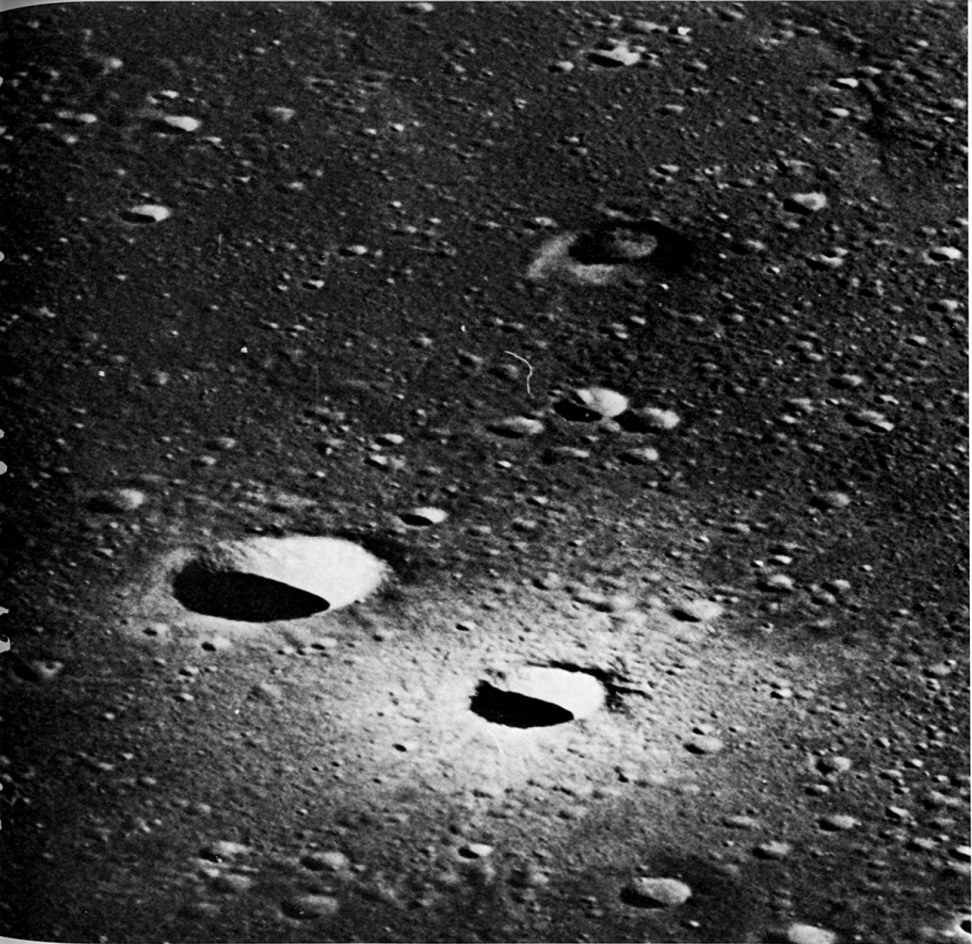
AS16-120-19237 (H)
FIGURE 88. - This is an oblique view of an area in Mare Nubium west of the central lunar highlands taken with the Hasselblad camera on Apollo 16. The small picture to the left is the entire frame reproduced slightly larger (112 percent) than the size of the original negative, and the larger picture on the facing page is a 12.5X enlargement of the area outlined within the small picture. The view shows two distinct types of lunar craters in the mare. The two craters in the near field are typical of a great many small impact craters. The larger one has a raised hummocky rim but no bright ejecta blanket and no visible rays. The smaller and probably younger one to the right displays an extensive hummocky ejecta blanket and a system of bright, radial rays. The dark crater near the middle of the photograph has little in common with the first two. It has a prominent, smooth, convex rim. It is not surrounded by a hummocky or blocky ejecta blanket and has no rays. These characteristics suggest that this crater is volcanic in origin. Occurrences like this indicate that both impact and volcanic processes were active on the Moon, and that they are both responsible for crater formation. However, as one can see in this view, impact craters are more numerous. F.E.- B.
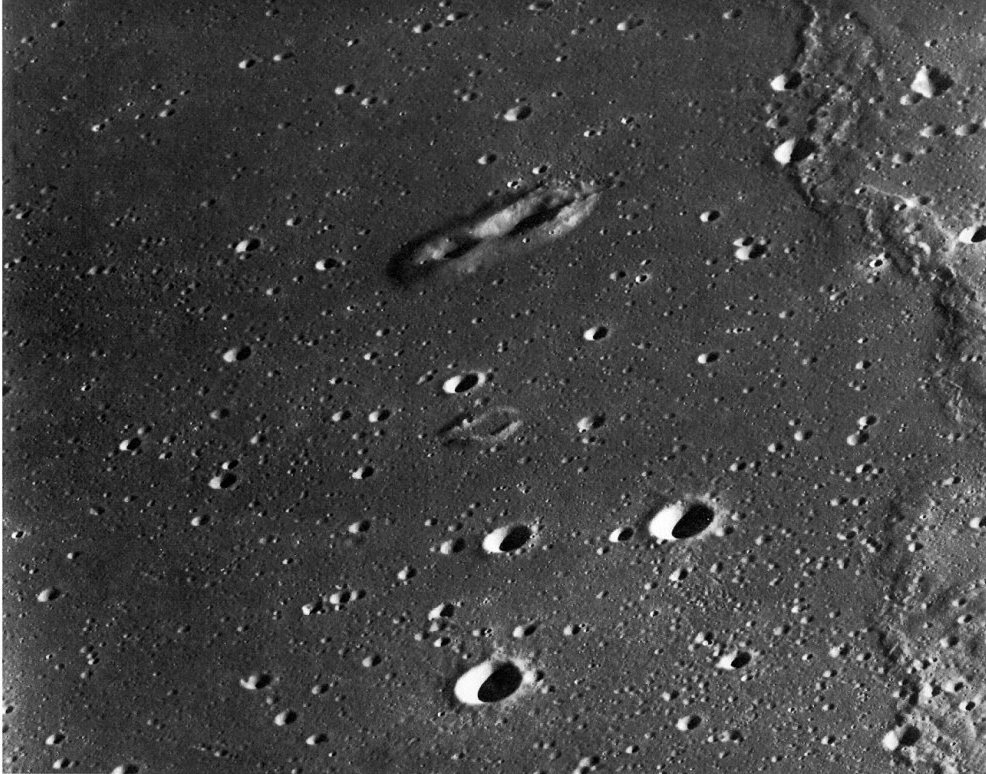
AS15-0344 (P)
FIGURE 89. - A few volcanic craters lie in a field of impact craters in Oceanus Procellarum, northwest of the Aristarchus plateau. Three in number, the volcanic structures are easily recognized by their high rims, planar slopes, and elongate shape. They are clearly embayed and partly buried by mare basalt, and thus are older than the mare plain surrounding them. - D.H.S.
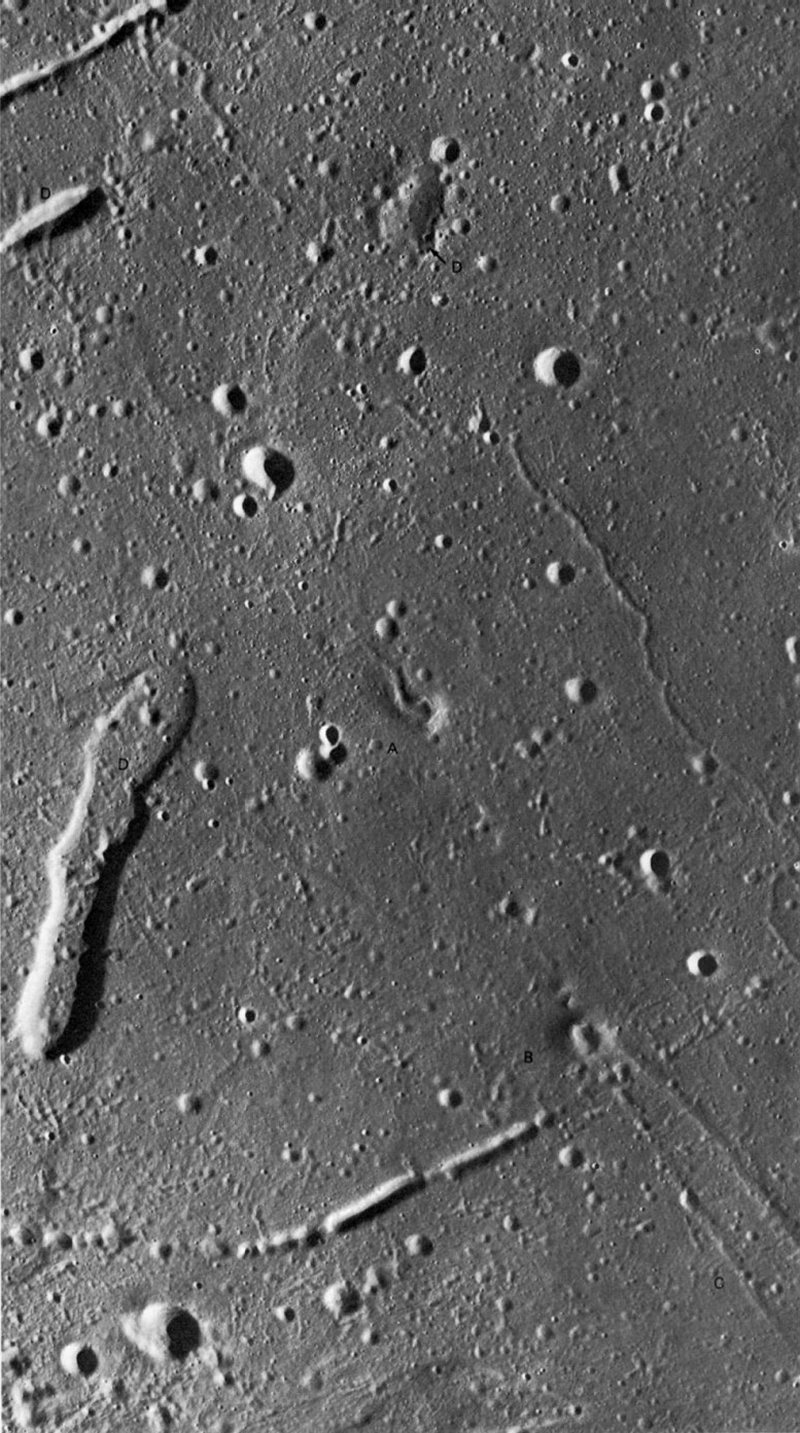
AS17-2317 (P)
FIGURE 90. - This panoramic camera enlargement shows two small (2 km across volcanic cones (A and B) near the southeastern margin of Mare Serenitatis. They are intergradational with the surrounding mare basalt and probably are similar in age (about 3 billion years). The same cones can be seen in their regional context in figures 58 (lower right) and 60 (lower left). One cone (B) has an enclosed summit crater and the other (A) has a breached crater leading to a rillelike valley. Both conditions are common among volcanic cinder cones on Earth. The cones appear to be alined along the buried extension of a straight rifle (C), suggesting a relationship between volcanism and tectonism that is common on Earth. In size, appearance, and geologic setting these lunar cones are remarkably similar to terrestrial cinder cones; by analogy they are probably composed of interlayered fragmental material and lava flows. The elongate depressions marked D, as well as the straight and arcuate rilles, are probably of structural origin resulting from faulting and collapse along fractures. - D.H.S.
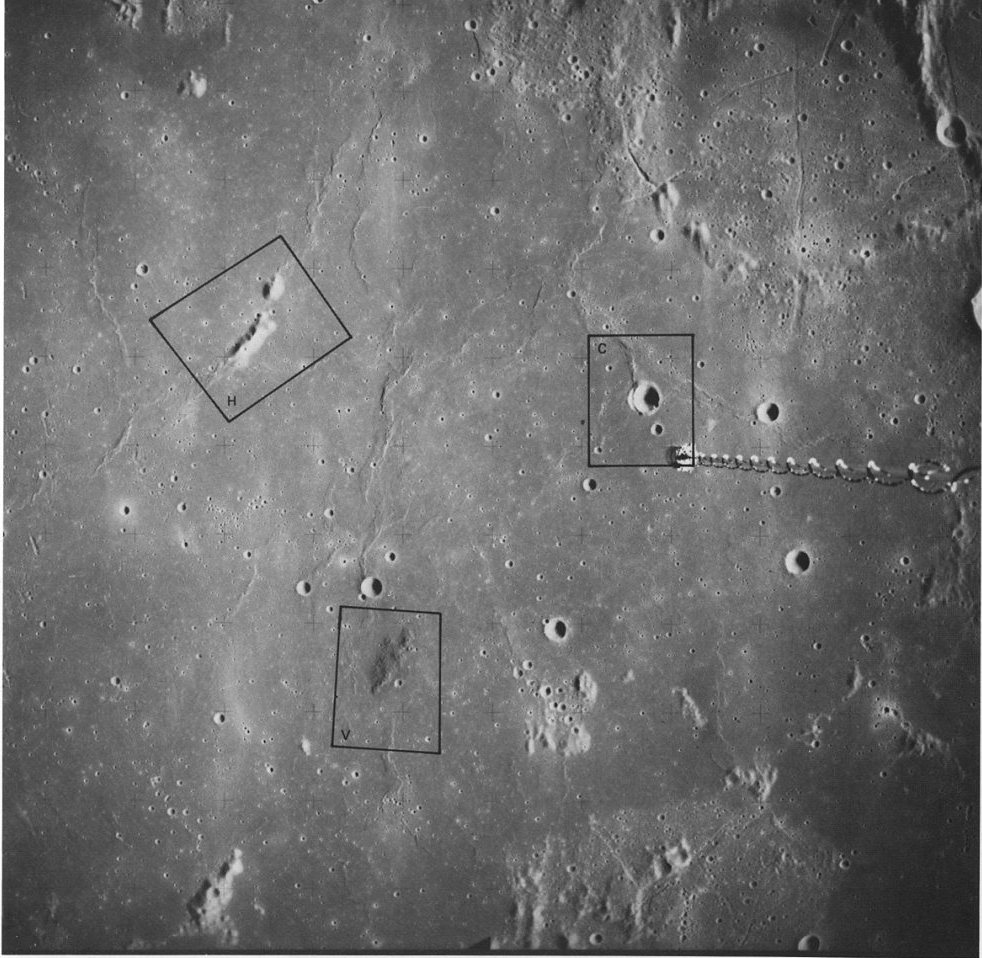
AS16-2824 (M)
FIGURE 91. - This picture of the eastern part of Mare Cognitum, while of interest in many respects, is used here largely to locate the dark hills (V) illustrated in detail in figure 92. Although small, the complex of dark hills is easily discernible because it is lower in albedo and is rougher than the surrounding mare lavas. Also included in this view are two bright hills (H) that were described earlier (fig. 69) as probably being of volcanic origin and an apparently faulted crater (C) that will be described later (fig. 246). Note the proximity of all three features to mare ridges. The dark hills lie within a westward bulge of one mare ridge, the two bright hills interrupt another ridge, and the crater is intersected by a third ridge. The association probably is not accidental. - M.C.M.
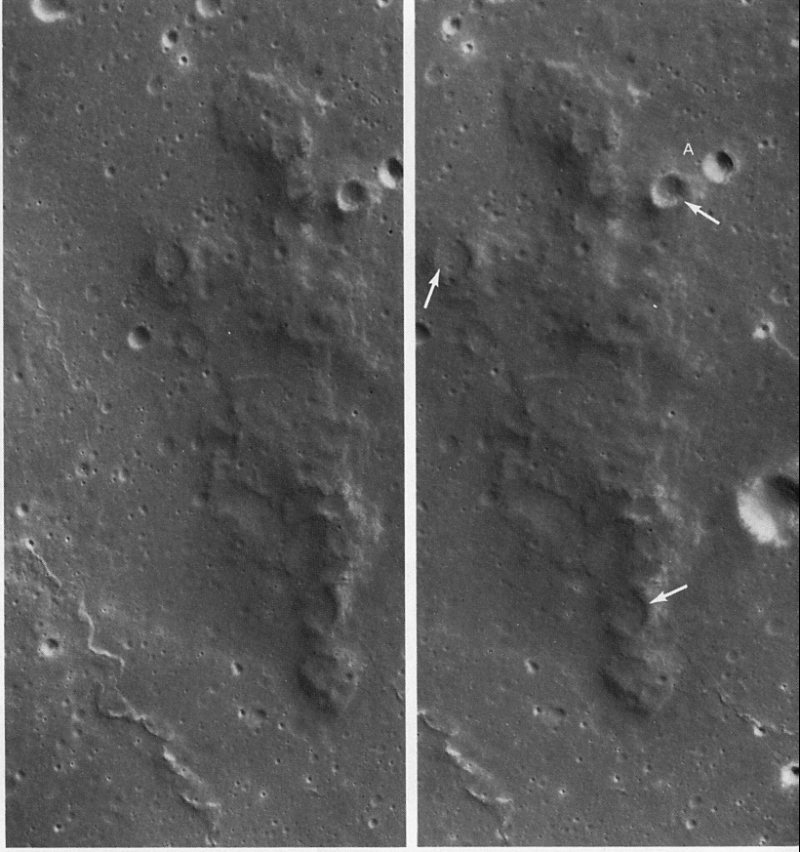
AS16-5433 (P) | AS16-5428 (P)
FIGURE 92. - The dark, rugged-surfaced complex of hills is enlarged many times in this stereogram. Without further detailed study, two explanations for its origin seem equally plausible. The complex may be a densely cratered block of terrae that was partly inundated by the lavas of Mare Cognitum and subsequently blanketed by dark volcanic ejecta. Within the darkened area, the concentration of fresh young craters is less than the surrounding mare surface, strongly suggesting that the dark blanketing was deposited appreciably later than the mare lavas.
Alternatively, the complex may be a pile of lava flows densely pockmarked by volcanic craters, and, as in the first case, subsequently covered by volcanic ejecta. The steplike, but discontinuous ledges along the east side of the complex probably represent successive flows of viscous lava. All the craters are shallow, probably because they have been filled by their own ejecta or by that from nearby craters. Nevertheless, several craters (see arrows) have steep raised rims, distinguishing them from impact craters. The difference is clear at A where a volcanic crater (left) can be readily compared to a normal impact crater (right). - G.W.C.
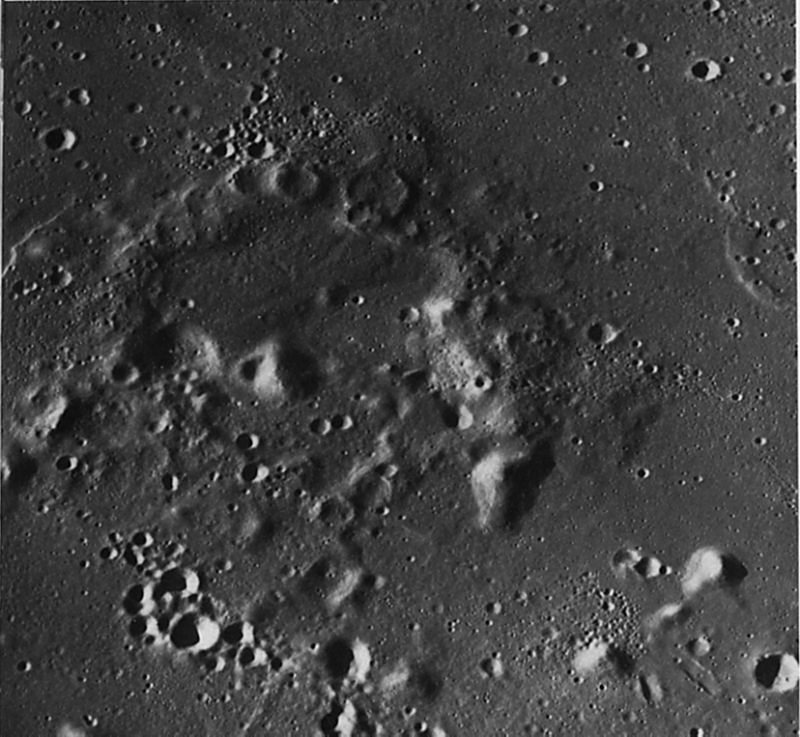
AS17-3114 (P)
FIGURE 93. - Easily identifiable volcanic complexes like the one in figure 92 are not common, but some others have been recognized. This crudely circular belt of high-rimmed craters is located along the common boundary between Oceanus Procellarum and Mare Imbrium, about 130 km southwest of the crater Euler. Here also, a cover of dark material-presumably volcanic ejecta-is present. However, unlike the previous example, which was surrounded by a "sea" of unbroken mare, this complex is closely associated with many peaks of premare terra. They are distinguished not only by their appreciable relief, but also by their smooth surfaces and lighter tone. - G.W.C.
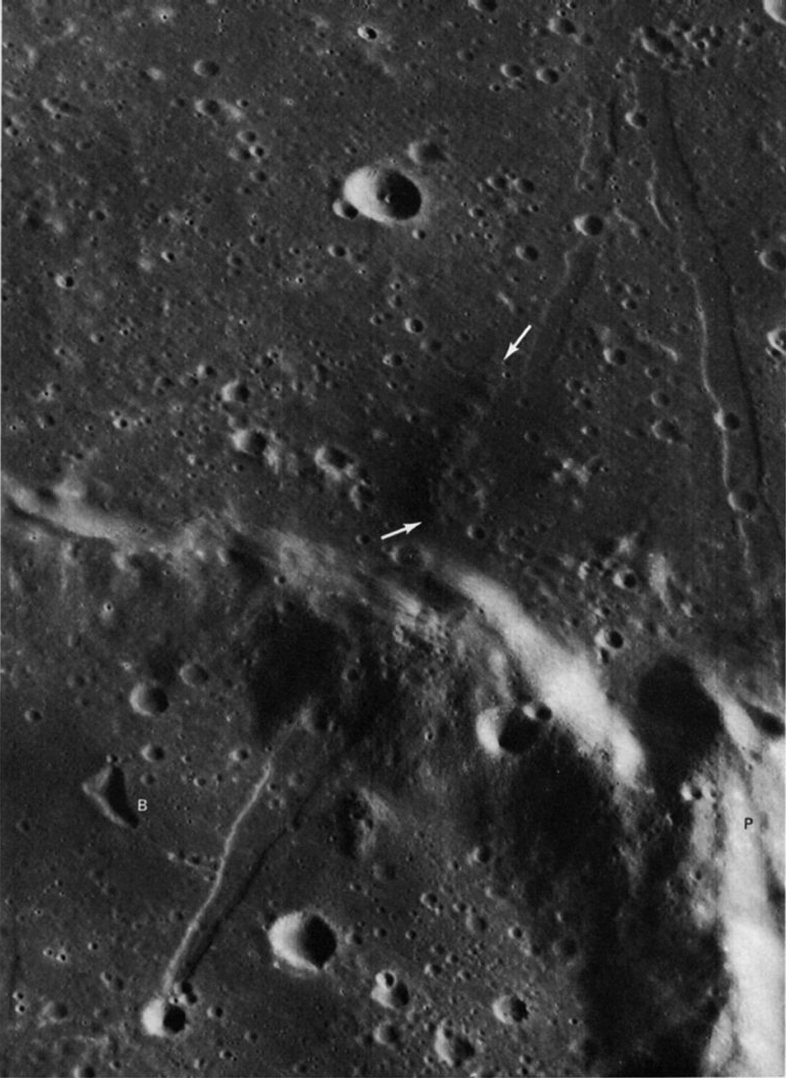
AS16-5425 (P)
FIGURE 94. - Patches of dark material-presumably volcanic ejecta-are commonly associated with straight rilles or grabens. This area is near the Apollo 14 landing site. (See fig. 44.) The prominent ridge separates the large ancient crater Fra Mauro in the north half of the scene from the smaller craters Bonpland (B) and Parry (P). The graben extending through the center of the picture cuts the floors of both Fra Mauro and Bonpland, as well as the intervening rim. Just north of the rim the graben has been completely filled and buried by dark materials. In appearance the low hills (see arrows) directly west of the graben resemble the volcanic complexes described in figures 92 and 93. The complexes are centered in the dark area and may well have been the source of the dark material. Starting with the formation of the three craters, the following events occurred: The craters were filled with mare lavas, the grabens were formed, and the dark volcanic materials were erupted. As everywhere else on the Moon, small bodies sporadically struck the surface throughout the entire interval of time. - G.W.C.
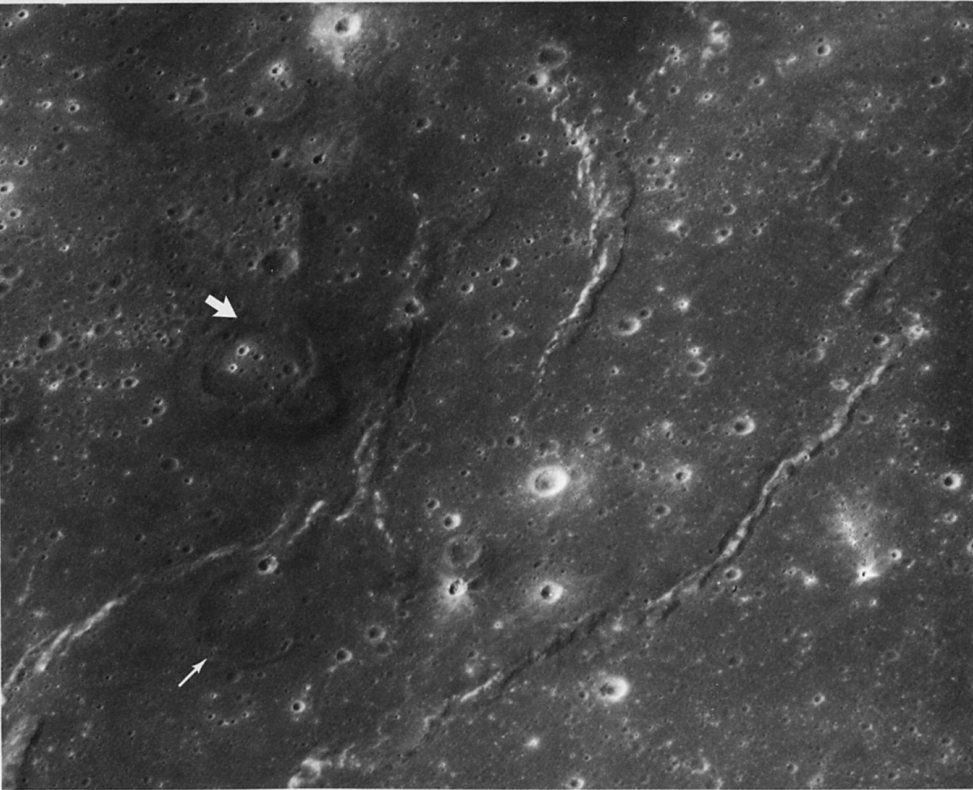
AS17-2224 (P)
FIGURE 95. - Resembling a dome whose top has collapsed, this unusual structure (see large arrow) may be just that. It is located in the southeastern part of Mare Crisium, a large mare-filled circular basin near the Moon's east limb. The mare ridges shown are part of an extensive ridge system that encircles the floor of the basin. The broad circular depression occupying the center of the dome may be analogous to the calderas that occupy the center of many volcanic edifices on Earth. It may have formed as the pressure exerted on a mass of molten rock being forced upward toward the surface was released, allowing the overlying rocks to settle. A similar appearing but smaller dome with a summit depression occurs on the northwest flank (lower right side as this picture is oriented) of the larger one. Another large circular structure (see small arrow) may have formed in the same manner, but the evidence is less compelling. It can just as easily be interpreted as being the remnant of a lava-flooded impact crater that was later blanketed by the dark material covering much of the area shown here. - G.W.C.