3. More, Better, Cheaper
If america managed to do so many things in space with expendable launch vehicles, as recounted in the previous chapter, why the Shuttle? The answer begins but by no means ends with the lowered cost of simply delivering payloads into orbit.
Freight rates for the Space Transportation System are based on recovering its operating costs and the U.S. investment in the Shuttle fleet and ground facilities, though not the original research and development expense, over a period of twelve years. Under the resulting price schedule, the Shuttle will place satellites in orbit for one- to two-thirds the cost of launches aboard the Delta, Atlas-Centaur, and Titan rockets used for most recent U.S. civilian and military missions and NASA launches for other countries. The expense of keeping up a varied inventory of launch vehicles and their different ground systems is eliminated. Based on traffic projections of more than fifty Shuttle flights a year when the system comes fully into use, the launch savings alone could be half a billion dollars a year or more, depending on inflation.
Since about 80 percent of the cost of space missions has been going into payloads, and only about 20 percent into launch costs, still bigger savings 30 to 40 percent of total payload program costs—will result from changes in spacecraft design made possible by the Shuttle’s great cargo capacity and by what it can do that one-way launch vehicles can’t.
Thanks to the Shuttle’s relatively gentle acceleration, designers of the spacecraft it carries may be able to use some off-the-shelf parts rather than creating and testing costly and rugged one-of-a-kind equipment. Because of the Orbiter’s large payload bay and great lifting ability twice that of the biggest expendable vehicle commonly employed- satellites can be simpler: less tightly packed, less limited in weight. Standardized parts and modular components may be used, and virtually the same spacecraft can be employed for different purposes by changing only its cameras or other sensors. The Shuttle’s ability to check out satellites in space while they are still in the Orbiter and again after they are deployed, to repair them in orbit, and to return them to Earth for overhaul also justifies designers in relaxing some reliability precautions, such as redundant circuits. This too saves money. Studies of how past spacecraft could have been designed differently if the Shuttle had been available to launch and service them showed that their costs could have been reduced substantially.
Still other payload savings are possible. Working models of instruments intended for long unattended operation in orbit can be deployed and left in space for weeks or months, then be retrieved and returned to Earth for examination and reworking if necessary. This will improve the reliability of future satellites and lengthen their lifetime at little cost while also reducing the time for development and ground testing. Prototypes of new instruments can also be tested in space for briefer periods while still attached to the Orbiter. Persistent problems can be pursued with early relights. Satellites can be retrieved at the end of their planned mission for refurbishment and reuse. Or modular components can be replaced in orbit, reducing out-of-service time, without bringing back the entire spacecraft. Satellites can be updated in orbit as technology advances, increasing their performance and prolonging their usefulness.
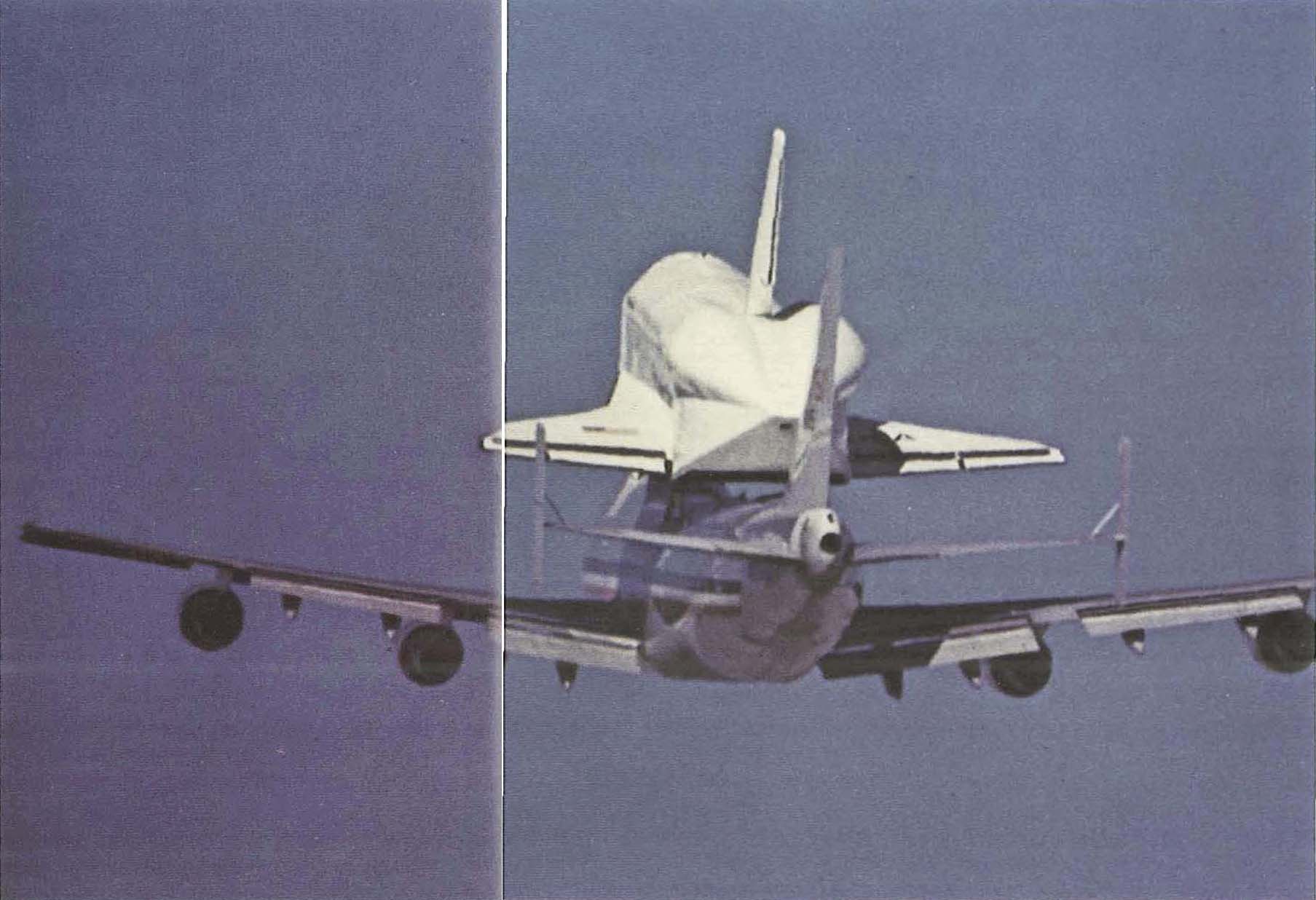
When all launches are made aboard the Shuttle and all spacecraft are designed to take advantage of its capabilities, there should be greatly reduced risk of costly total failures in spacecraft operations. Even a failure of the Shuttle vehicle itself need not be catastrophic: the crew could carry out one of the abort procedures described in Chapter 7, landing safely with the payload intact. A study of 131 failures of the 1960s and ’70s found that seventy eight of them, related to the launch or to malfunctions early in the mission, could have been detected during checkout in the Orbiter or just after deployment. They were problems that could have been corrected immediately or by bringing the spacecraft back to the launch site for repairs. The other fifty-three were later failures or erratic behavior that could have been corrected by retrieving the satellite for repair and relaunch.
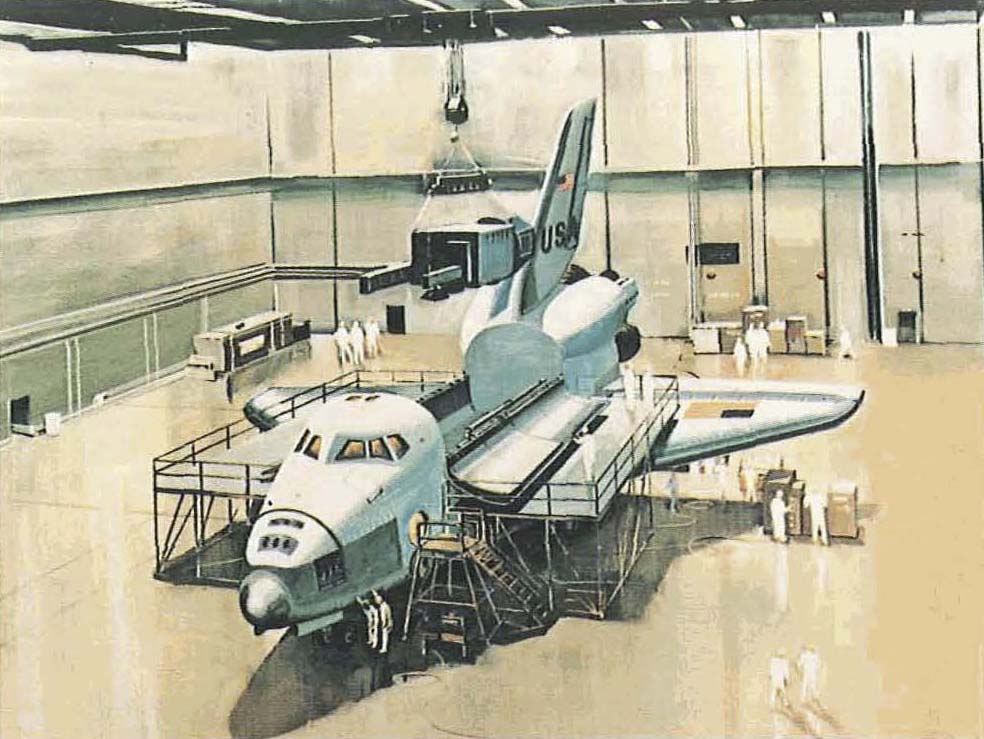
An interesting example was cited by the deputy director of NASA’s Shuttle Program, LeRoy E. Day. Two out of three Orbiting Astronomical Observatories launched between 1966 and 1970 suffered fatal mishaps. A battery charger failure after two days on the first OAO could have been corrected by returning the satellite from orbit and repairing it. The third one failed to reach orbit because the shroud that protected it during launch wasn’t discarded at the right time; this would have been avoided if the Shuttle had been the launch vehicle, since the spacecraft would have been carried inside the Orbiter and have needed no shroud. The second OAO performed beautifully, but even the problems that occurred beyond its planned operating lifetime could have been corrected in orbit, extending its service, if the Shuttle had been available and the spacecraft had been designed for such maintenance.
The Shuttle has other unique virtues. It can be prepared for launch on relatively short notice. Thus it could conceivably carry out a rescue mission, one Orbiter bringing safely home the crew of another Orbiter disabled in space and unable to return. It can be sent off quickly on a special mission to gather information needed in an emergency on Earth, such as a flood or crop blight. It requires no elaborate and expensive operation at sea to recover the crew after each mission, as in Apollo and other past manned flights. And it can take scientists and engineers into space routinely to conduct their own experiments and observe the results first-hand, to test instruments of their own design and make immediate adjustments. The mission plan can be changed during a flight to cope with problems or take advantage of opportunities.
Both the Orbiter’s large weight and volume capacity and the price schedule for flights encourage use of the Space Transportation System by a wide variety of customers. A number of spacecraft can be carried on the same flight, and sharing by different users is encouraged. Experiment packages intended to remain within the cargo bay can be fitted in with primary payloads. To stimulate early use, rates are frozen for the first three years, and are the same for U.S. and foreign commercial firms; later they will be adjusted annually on the basis of operational experience. A user that books a full flight and finds there is some room left over can sublet it. Discounts are offered to shared-flight customers who agree to fly their payloads on a space-available (standby) arrangement. Applicants who propose an exceptional new use of space or a first-time use of great potential value to the public are considered for special rates. NASA has established a small technical group to advise and help people with little or no experience in space research.
Small self-contained experiments will be flown as standbys for $3,000 to $10,000 (in 1975 dollars), depending on size and weight. Of the first 250 reservations, a quarter were for educational purposes and a fifth were from individuals planning to test new concepts in the space environment. Some were donated to high school and college students developing experiments as part of their academic work. Fifty-one were from foreign countries: Germany, Denmark, the United Kingdom, Canada, Japan, Israel, and Egypt. The payloads must be for research or development—no commercial gimmicks. Said the director of NASA’s Space Transportation System Operations, Chester M. Lee: “We have had to turn away a few speculators who wanted to send up hunks of metal and later sell pieces as souvenirs or who wanted to send up postage stamps and sell them at high profit.”
By early projections, about half of the payloads will be for NASA, about a fourth for the Department of Defense, and the rest for other U.S. government agencies, U.S. private organizations, and foreign governments or companies. Civilian users, large and small, are expected to include communications networks, research foundations, universities, observatories, state agencies, county and city planners, public utilities, farm cooperatives, medical research groups and health services, the fishing and transportation industries, oil and mining interests, manufacturing and aerospace firms, chemical and pharmaceutical companies, water conservation and power generating authorities, and private citizens. Developing countries can begin space programs of their own at affordable costs by sharing Shuttle missions with other users and flying modest payloads that are exposed to space from the Orbiter, then later perhaps move up to more ambitious projects like domestic communications satellites.
The returns from America’s investment in the new Space Transportation System, then, come not only from reduced launch, spacecraft, operations, and man-in-space costs. They will come also from both the increased and wider use of space stimulated by ready access to reliable, frequent, flexible, economical two-way freight and passenger service between Earth and orbit. And they will come, ultimately, from new ways of using space -including uses not yet thought of.
NASA Administrator Robert A. Frosch spoke to a committee of Congress about changes in the basic approach to space flight:
For twenty years we have had to reach for the benefits of space in small, expensive, prepackaged increments. Each mission has been such an increment, with its long lead time, one-way transportation system, weight and volume constraint, demands for redundancy, extraordinary test rigors, and conservative failure margins. . . . The early Shuttle missions we will see are relatively straightforward evolutionary extensions of present approaches. . . . It takes time before we recognize that we can afford in space an approach to experiments similar to those we use on the ground, because of the easy presence of the human experimenter, supervisor, technician, or repairman. We will have to stop thinking in terms of discrete space missions, each with its own spacecraft, its own control center, its own ground network, its own clientele. . . .
In parallel with the changes in how we will be operating in space are the implications for what we can do there. Perhaps the best current example in science is that of the space telescope. It is important to realize that astronomers have been planning for the telescope since the early ’60s-and that only the advent of the Shuttle revisit for orbital maintenance has made it practical. The space telescope is our first facility in space, now not much more remote from human attention than the more limited instruments we have built on mountain cons around the world.
We are at the beginning of another revolution today as well: one in communications. It would be appropriate to term this the “second communications revolution.” since the satellite developments of the past fifteen years have already completely changed domestic and international point-to-point telecommunications traffic. . . . Just around the corner. . . .is the next quantum jump in this field. . . .
The geometry of the world and the space around it, coupled with the technological capability to build large antennas and supporting facilities in space while vastly simplifying and reducing ground terminal size and complexity, make the possibility of hemispheric interconnections at the “CB” level a reality. Concepts of public service telecommunications like electronic mail, medical information service delivery, continuing interactive education, and broadly based information access now await implementation decisions rather than technological feasibility demonstration.
Designers of commercial communications satellites are studying concepts for future ones that would be four times heavier than today’s and twice as bulky. The larger designs relieve many of the problems of packaging antennas and providing more area for solar cells for increased power. They should be more efficient and more reliable, and provide additional channels.
Frosch spoke also of a “global information system based on remote sensing of the land, sea, and air from satellites launched and maintained by the Shuttle. “I think that rather than having individual satellites for individual purposes,” he said, “we will more and more see ourselves as building a multipurpose system of satellites and sensors, with the means for broad-scale data transmission and archiving and processing data into information. This type of system would look at all features and characteristics of the entire surface of the Earth and its atmosphere that can be sensed from space. With this versatility, we would be able to provide a variety of data to different users.”
High-resolution remote sensing of natural resources from space will extend uses of such information that were demonstrated during the 1970s (Chapter 2). Instruments aboard Orbiters and a new generation of Earth-looking satellites will be able to detect crop and timber diseases and insect plagues, map ocean currents and temperatures that affect the movement of fish, maintain a worldwide watch on air pollution, provide information for experiments in weather modification and better understanding of climate, and forecast global production of several food crops.
The Shuttle also permits researchers to extend their investigations of how the unique conditions in space-virtually no gravity, near-perfect vacuum, very low vibration—might be used in manufacturing products that are difficult or impossible to make on Earth. Some ideas were tried out in Apollo, Skylab, and Apollo-Soyuz and in brief rocket flights with promising results. Government, university, and industrial scientists are planning both untended and hands-on experiments in Spacelab (Chapter 8). Most of these seek to take advantage of the lack of gravity. In the weightlessness of space, liquid mixtures of materials of different densities can be solidified without separating, as they would on Earth, by the heavier ones sinking to the bottom. Liquids may be floated freely during processing without being contaminated by reaction with containers. Large, flawless crystals can be grown without being distorted by their own weight as they form. Future possibilities for commercially valuable produces include composite materials and metal alloys, electronic and optical crystals, new kinds of glass, and biological materials for medical research and treatment.
An entirely new activity seen as possible with the Shuttle is the building of large structures in space. Size and weight need no longer be limited to the payload of a single launch vehicle. A series of Shuttle flights could deliver structural members or modules to orbit for assembly there. An Orbiter could serve the construction crew as living quarters as well as provide electrical power, communications, and data processing. One or two electro-mechanical cargo-handling arms, attached to the Orbiter, could assist the space-suited builders in moving large pieces into place. Because there’s no gravity, space structures could be very large yet relatively flimsy without collapsing of their own weight. Structures that might be assembled 27 in space include large communication antennas, solar energy collectors and transmitters, manned laboratories, processing and manufacturing facilities, large spacecraft assembly plants, warehouses, and refueling and repair depots. One day a Shuttle may build an advance base for an expedition to the farther shores of the solar system.
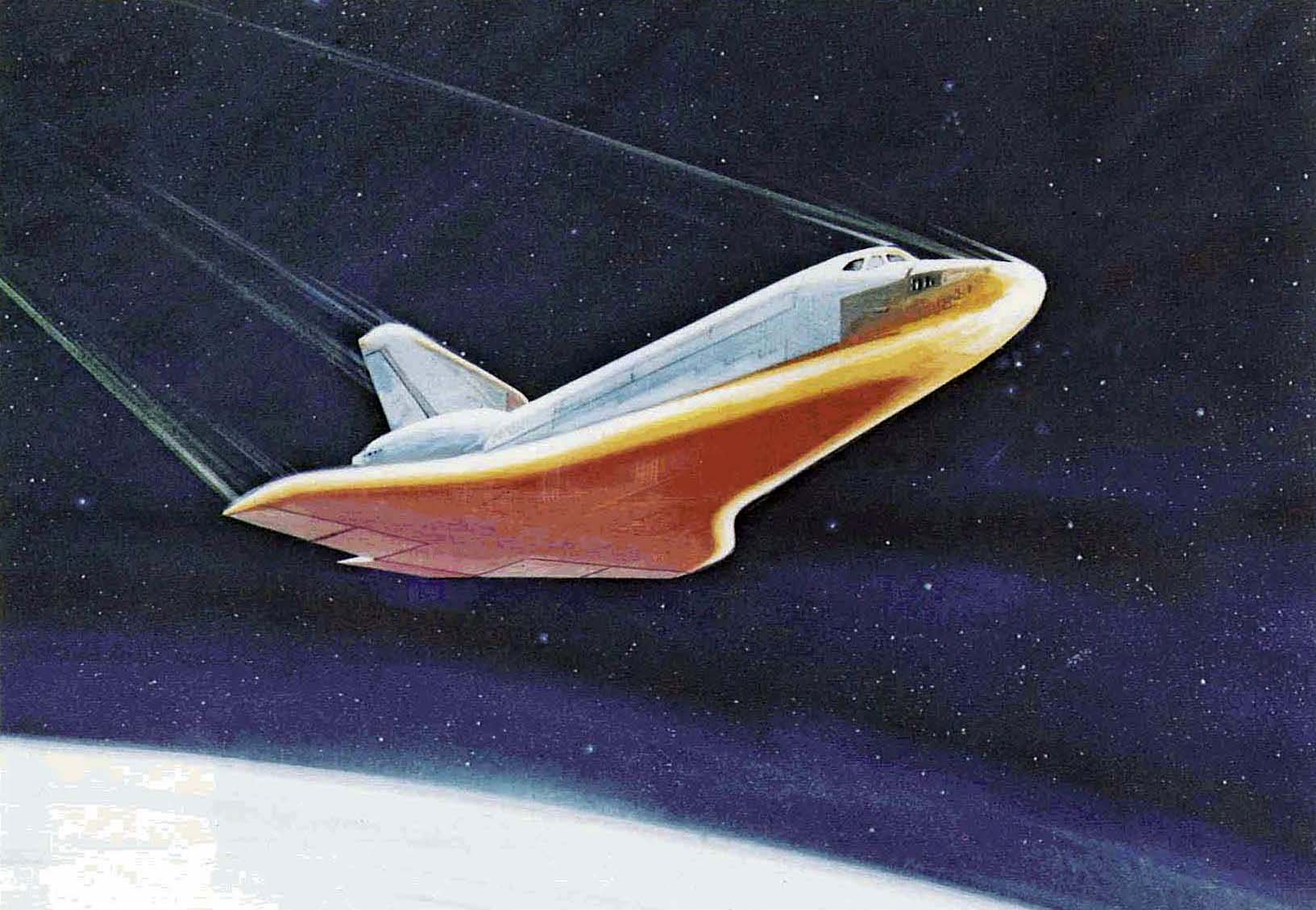