9. Plans, Possibilities, and Dreams
The shuttle is planned as the key element of American operations in space through the 1980s and into the ’90s. What can we expect in growth of its abilities and extension of its uses? NASA is necessarily conservative in making firm plans, limited by budgets and expressed user needs, but is imaginative in the range of possibilities being examined. Some of the ideas discussed here may startle anyone but a reader of science fiction. All, in fact, have been looked at soberly by NASA planners or other hard-headed engineers, scientists, and economists in the Government, industrial companies, and universities.
NASA’s advanced studies envisage an evolutionary buildup from longer Shuttle flights to free-flying Spacelabs, automated and then manned orbit to orbit freighters, Shuttle-tended and then continuously occupied space bases, demonstrations of solar power generation and other industrial applications of space, and wide personal use of space technology like electronic mail delivery and wrist telephones linked by satellite.
The first step is to extend the duration of the Orbiter’s flight beyond the current capability with extra tanks of propellants for its attitude-control and maneuvering engines and of hydrogen and oxygen for its power-generating fuel cells. One solution planned is a utilities module that is carried to orbit in the payload bay and left in space. It will unfurl large winglike arrays of solar cells to collect sunlight for production of electricity, generating twenty-five kilowatts of power for the Orbiter and experiments on board. It will also contain extra payload-cooling radiators and a set of gyroscopes for attitude control of the Orbiter and attached payloads. The gyroscopes save maneuvering fuel by eliminating the need for frequent firing of the control thrusters.
A twenty-five-kilowatt module can supply power for a Spacelab or construction mission of sixty days or more. After sixty days the flight would be limited by such factors as food and drinking water. The module could also supply plug-in power for free-flying payloads that would dock with it, and it could be detached and parked in orbit between Shuttle missions. One version could itself fly free of the Orbiter with instruments for, say, studying the Sun or Earth. Another could be attached to a free-flying Spacelab for long-duration missions like observing the Sun continuously through two or more 28-day solar cycles or studying plants or animal specimens through several generations. A Spacelab with its own utilities and attitude-control module could operate a long time a step toward a permanent space station- if resupplied periodically by the Shuttle with food, water, and other consumables.
NASA planners foresee needs for considerably more than twenty-five kilowatts of power in space by the middle or later 1980s:
- About fifty kilowatts for a multibeam communications satellite serving hundreds of thousands of very small receivers on Earth wrist telephones- or a prototype materials-processing laboratory if early Spacelab experiments prove promising;
- A hundred kilowatts for electronic mail—nearly instantaneous facsimile transmission of letters and other documents through satellites and more for lesung space-to-underwater communication;
- Two hundred and fifty kilowatts for a Shuttle-tended space base to construct a large precision antenna or for a low-orbit space-power test project. This could evaluate power transmission efficiency, pointing accuracy, possible heating of the atmosphere, and other factors to be considered before proceeding with a large-scale, high-orbit plant to collect solar energy and beam it to Earth;
- Several hundred kilowatts for solar electric propulsion for moving large objects from low to high orbits or for some of the exciting long-duration scientific missions like rendezvous with a comet or flybys of the outer planets.
This power could be generated by nuclear reactors or by the Sun. For both technical and environmental reasons, NASA so far prefers solar power. The Sun can be used to drive rotating generators; to convert solar heat directly into electricity with thermionic systems; or to convert the Sun’s electromagnetic radiation into electric current with photovoltaic cells. These, commonly called solar cells, employ a semiconductor such as silicon that releases electrons when bombarded by photons from solar radiation. Because of its successful experience with solar cells for many years to power scores of satellites, NASA favors continuing with them; it has devised several configurations of an enormous 250-kilowatt photovoltaic power module.
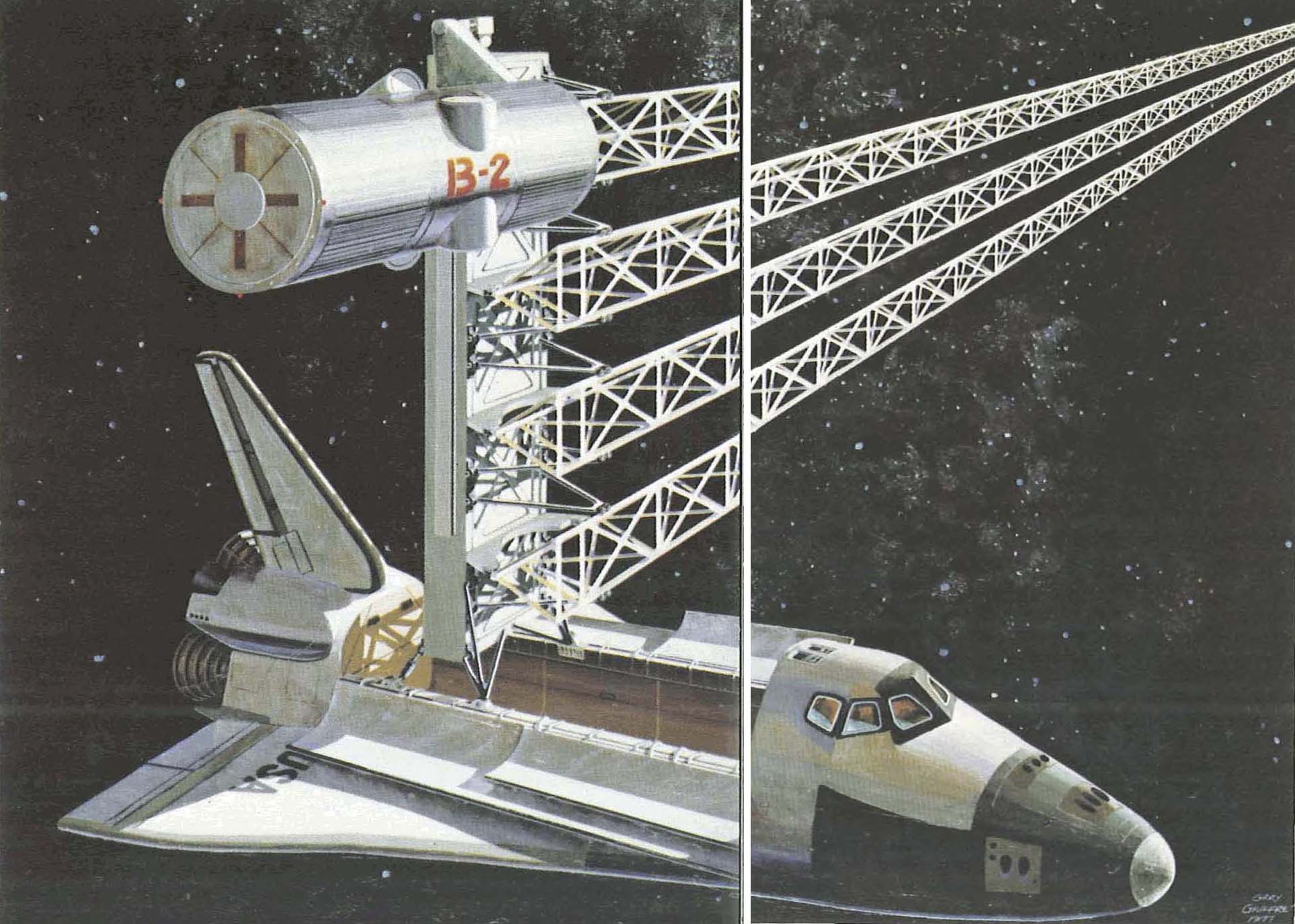
Preparing to build and use a solar power generator of that size or larger, with dimensions in hundreds of meters, requires advances in a lot of other areas: transportation systems, cranes and remote manipulators, jigs and fasteners, so-called cherry pickers like the ones that hoist powerline repairmen, power tools that can be handled by construction workers in space suits. A free-flying robot tractor designed in the 1970s for moving large objects around in space-NASA calls it a teleoperator—can be used not only to assemble space structures but also to place Shuttle payloads in medium-altitude orbits beyond the Orbiter’s range and retrieve them for servicing.
For very large structures, it will make more sense to fabricate some sections in space rather than bringing up pieces in the Shuttle for assembly. An aerospace company has developed an automated beam builder to fit in the Orbiter that can extrude triangular girders from compact coils of ultralight metal plate. It’s fed through rollers that shape it to the desired cross section in a manner similar to the on-site fabrication of aluminum rain gutters. The beams would be so light-less than a hundredth the weight of comparable ground construction—that a single Shuttle flight could bring up material for a structure approximately 300 by 100 by 15 meters (1000 by 325 by 50 ft).
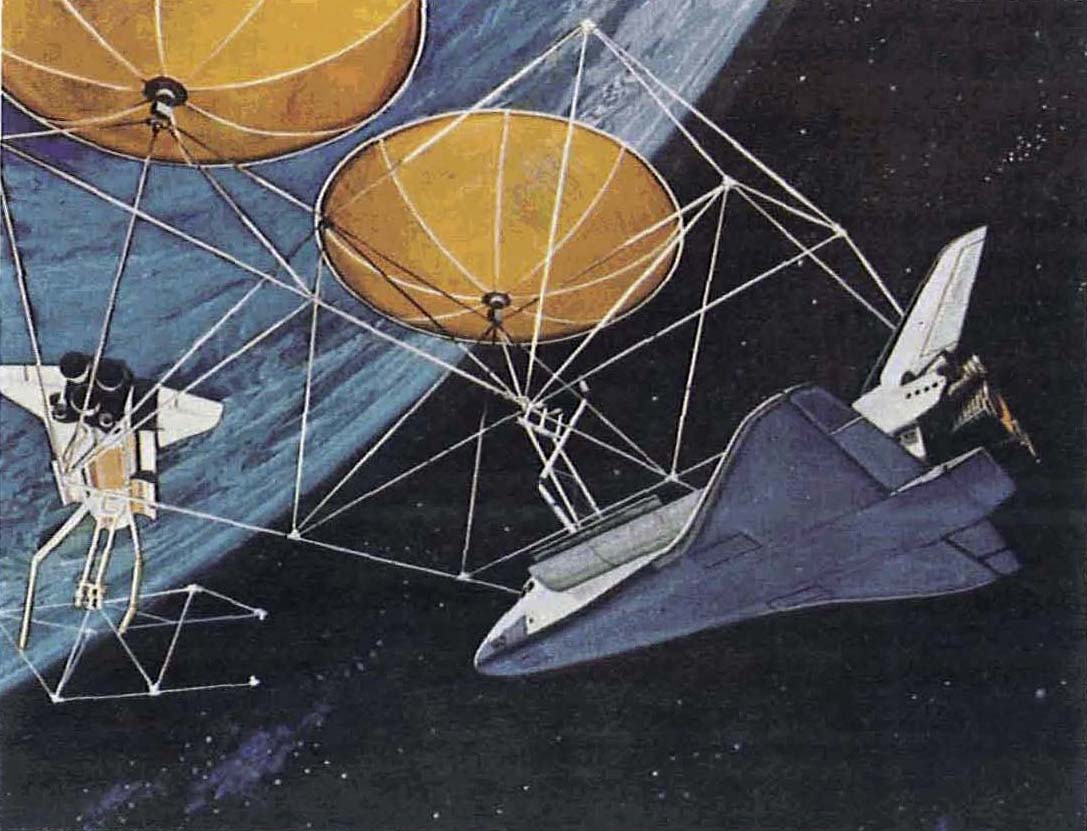
A number of large space structures of the future, including multipurpose communications platforms, must operate in geosynchronous orbits in order to provide continuous coverage of their service areas. They not only will outgrow the size and weight capacity of the first-generation Shuttle-launched upper stages described in the previous chapter but also will require servicing in orbit to extend their working lives and spread their high original costs over many years. Some may be so large solar power stations, for instance-that they can best be built at their operating sites. Hence a need for reusable, manned vehicles to carry both cargo and work crews between low and high orbits. A two-stage hydrogen-oxygen orbital transfer vehicle could be assembled in low orbit from separate stages carried by two Shuttle flights. Other Shuttle flights would bring up the propellants, cargo, and crews.
To avoid the complications of loading propellants in orbit, however, NASA planners are studying ways to increase the Shuttle’s lift. A growth from the present limit of 29,500 kilograms (65,000 lb) to about 46,000 kilograms (100,000 lb) would allow two upper stages, already fueled, to be carried to low orbit by two Shuttle flights and linked there. One concept for a low-cost, heavy-lift launch vehicle uses the Shuttle’s solid-propellant launch boosters and its three hydrogen-oxygen main engines, attached to the big fuel tank, but substitutes a large payload, covered only by a light protective metal shroud, in place of the more costly Orbiter. A single launch could then put more than three times the Shuttle’s present maximum load in low Earth orbit. (Still mightier launch vehicles proposed by aerospace companies would team up sixteen or twenty-four engines to lift payloads of 225,000 or 275,000 kilograms (500,000 or 600,000 lb).)
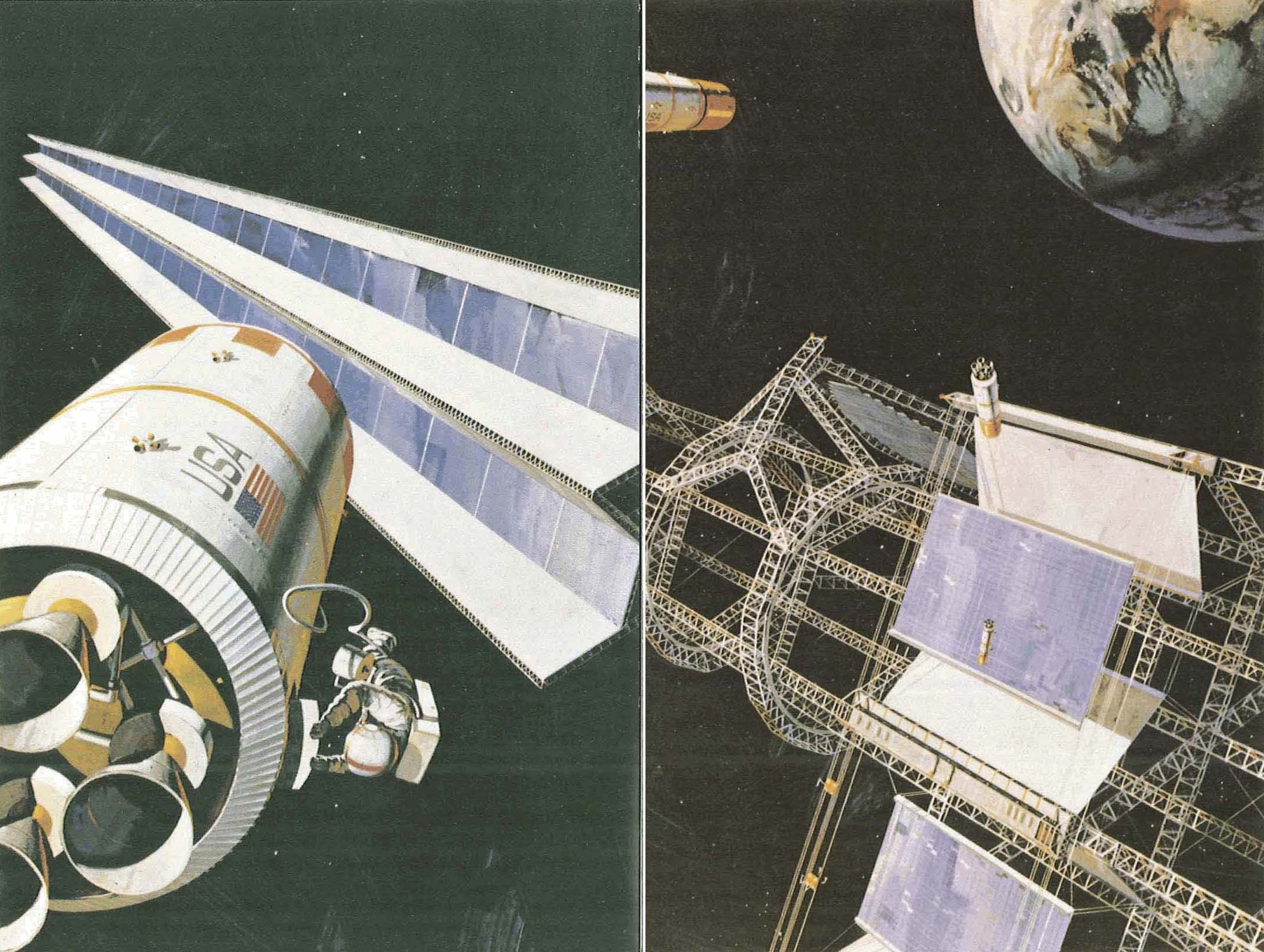
Looking beyond NASA’s recent studies of possible future space operations, the agency’s director of Advanced Programs, John H. Disher, predicted in an article published as the Shuttle was being prepared for its first orbital test flights: “. . . the Shuttle and Spacelab, I believe, will energize space flight as the DC-3 and DC-4 did aviation-prompting greatly increased use of the unique features of space both for applications we understand today and for applications not yet conceived. Given this spur, I can see advances being made substantially more rapidly than provided for by our current quite conservative plans.”
Energy shortages and pollution worries, and the prospect of worse to come, have focused special interest on the idea of converting space sunlight- -unlimited, unfiltered by the atmosphere, uninterrupted by nightfall into electricity for consumers on Earth. The respected American Institute of Aeronautics and Astronautics (AIAA) selected space power, along with materials processing (discussed in Chapter Three) and life sciences research (such as gravity-free bed rest for treatment of burns and fractures), as three particularly promising future ways to use the unique environment of space to help solve problems that are complicated by gravity and the atmosphere.
While noting that more research will be needed to establish the economic feasibility of space power, the AIAA study said, “There is little question of technical feasibility: all elements of prospective power plants have been established by either experimental tests or long periods of operation in space. ...” The report listed a number of advantages besides ample free sunshine for locating power plants in orbit: isolation from populated places, no earthquake hazards, easy disposal of excess heat, savings of natural resources by lightweight construction, no corrosion of materials, no pollution, no need for energy storage or backup facilities.
The AIAA committee considered two ways of generating power: immense arrays of solar cells and large collectors of solar heat. The collectors would focus sunlight on a central receiver, heating a gaseous working fluid to drive a turbine, compressor, and generator. Either type of station would beam the energy to Earth as microwaves, which would be collected by large antennas and converted to alternating current for distribution by ground power grids. The generating plant, the study said, might be assembled in low orbit from components carried by future heavy-lift vehicles and then be moved to geosynchronous orbit by electrical thrusters using power generated by the plant itself on the way up.
(The report also mentioned as “extremely interesting” a proposal, originally suggested by proponents of space colonization, for building space power plants from materials mined on the Moon. The material would be refined and structures fabricated in solar-powered factories at neutral-gravity locations between Earth and the Moon. Cheaper transportation than from Earth, thanks to the Moon’s low gravity, would offset the cost of the lunar mining base.)
The AIAA study suggested that a solar power system of several generating stations, though it could cost tens of billions of dollars, might be paid for while being built up over several years from the sale of power at prices competitive with ground-based plants. “As a nonpolluting limitless source of energy,” the report said, “space-based solar power stations could lead to a system capable of producing much of the United States’ power requirements early in the 21st century, and in the very long term could conceivably develop into the world’s primary source of electric power.
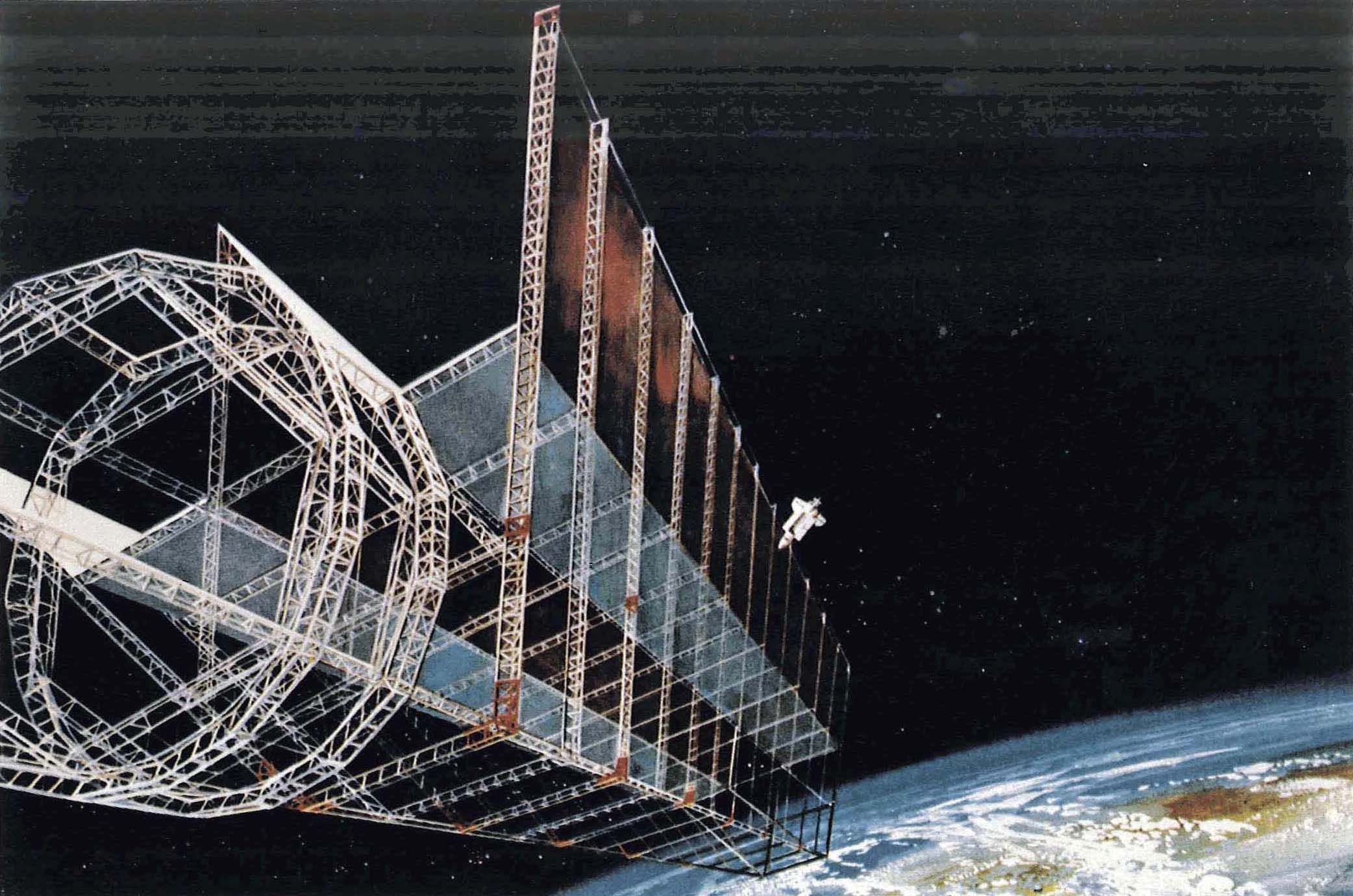
The Government’s position is more cautious. “It is too early to make a commitment to the development of a satellite solar-power station or space manufacturing facility, due to the uncertainty of the technology and economic cost-benefits and environmental concerns,” a White House statement said in 1978, then continued: “There are, however, very useful intermediate steps that will allow the development and testing of key technologies and experience in space industrial operations to be gained. The United States will pursue an evolutionary program that is directed toward assessing new options. ...”
Aerospace company officials, understandably, see grander visions. One said his firm has identified 150 opportunities for profit-making space industrialization, including chitty-five for space manufacturing of new or improved products ranging from pharmaceuticals to high-strength permanent magnets. He envisages extremely large multibeam antennas in space making possible pocket telephones and also electronic tele-commuting: “Rather than driving to work each day, the workers would operate from their homes or from a small satellite office where they could interact electronically with people and machinery in a central office building in a nearby city or in one located many hundreds of miles away. This . . . would help solve our energy problems and improve efficiency. It would also allow a life-style whereby people could live, work, and play in small communities, but still perform jobs that are essentially urban.”
He cited a study which estimated that industrial uses of space could create 100,000 new direct jobs by the mid-1980s and nearly two million by the year 2010. Through the multiplier effects, the study forecast, this could lead to two or three times as many total jobs and an increase of hundreds of billions of dollars in the gross national product.
Others dream of space tourism: a NASA consultant sees a 100-room hotel by the year 2000 with rates-presumably not for the average family vacation-starting at $5000 for the round trip and a few days in orbit. And of permanent settlements in space. In an exercise in realistic imagining, a group of engineers, architects, physical and social scientists, and others met for ten weeks in the summer of 1975 at Stanford University and the nearby NASA Ames Research Center and designed a city in space for 10000 inhabitants. The AIAA assessment of future practical applications of space, in discussing the potential of a life sciences laboratory, said: “It is almost certain that studies on plants will lead to being able to culture plants for space colonies and that these plants will be able to use human waste products to generate food and oxygen.”
Dreams?
An economist who has done several cost-benefit studies for NASA on other subjects: “The establishment of space habitation will be an evolutionary outcome of the current United States space program. Mankind will achieve in the next 100 years the most significant accomplishment yet: true Earth. independent, self-support systems which will lead to the establishment of a multitude of new, different, and enterprising civilizations.”
And John Disher, in his article on NASA’s own advanced studies: “No one can foretell when we may have permanent settlements of people in space or large-scale use of resources from the Moon or asteroids for space construction. The benefits, costs, and risks of such undertakings remain to be established. Fortunately, however, the nearer-term developments discussed here will proceed on their own merits and constitute necessary developmental steps toward the longer-term possibilities...”
Possibilities…?
Dreams
Or goals?
Time will tell. Decades from now some of these ideas may seem innocently unrealistic, based on ignorance of hard reality. But it’s also possible that some will seem astonishingly timid, cautious forays by limited imaginations. (One remembers those 19th Century visions of future air travel in ship staterooms aboard sail-driven balloons.) There may be as much chance that we will undershoot as overshoot in predicting the topography of the future.
What we are concerned with are not solely engineering measurements like mass and specific thrust. Fully as important is another kind of thrust: the questing human spirit.